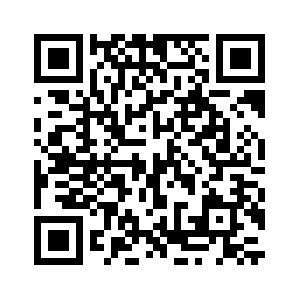
A New Physical Phenomenon Discovered When Microbiology Meets Surrealism: The Yoshida Effect has the Power to Fuse Bacteria and Nano-Acicular Materials
Microbiology受け取った 22 Jul 2024 受け入れられた 30 Jul 2024 オンラインで公開された 31 Jul 2024
Focusing on Biology, Medicine and Engineering ISSN: 2995-8067 | Quick Google Scholar
Next Full Text
Analysis of Reliable Transmission Performance Optimization Methods for Satellite-to-Ground Laser Communication Links
Previous Full Text
Mars Ascent Propellants and Life Support Resources - Take it or Make it?
受け取った 22 Jul 2024 受け入れられた 30 Jul 2024 オンラインで公開された 31 Jul 2024
Surrealism is a means of artistic expression that places automatism at the root of creation, and it has pursued thought that is entirely free of any preconceived notions or restraints. Art and science are seemingly incompatible with each other—one is emotional, the other rational—but here the author would like to consider the sort of thinking that could emerge if science met surrealism halfway. The author would also like to present the Yoshida effect, a physical phenomenon that was chanced upon serendipitously in which microbiology approaches surrealism. The Yoshida effect is the formation of a fusion body called a penetron when bacterial cells collide with a nano-sized acicular (needle-shaped) material in a hydrogel friction field. The penetron as an intermediate was applied to the finely detection method of asbestos, and gene transformation method by plasmid DNA.
Surrealism is an artistic form that developed in the 1920s. The assertion of Surrealism tends to be misunderstood as an unreality beyond reality, but in fact, it refers to reality that has been taken to excess, or super-reality. The surrealist artists aimed to create a greater, more profound surrealism by mixing reality and dreams. In his 1924 “Surrealist Manifesto,” the writer André Breton defined surrealism: “Pure psychic automatism, by which one proposes to express, either verbally, in writing, or by any other manner, the real functioning of thought. Dictation of thought in the absence of all control exercised by reason, outside of all aesthetic and moral preoccupation” [
].The origins of surrealism may be seen in Dadaism [
], and its thinking is rooted in automatism. Surrealism proposes to bring into view an inner psychological world that humankind has so far not experienced. Is it possible to fully strip away the consciousness of the subject and thus make assertions through only the object? Can there be such a thing as unconscious consciousness? André Breton was aware of the uncanny ability of the human brain, and he attempted to apply this ability to art. Research to date has shown that surrealism can be found even before Breton’s discoveries in Renaissance paintings [ ], novels [ ], and poems [ ], suggesting that Surrealism may perhaps be a human ability to generate creativity.Surrealists have been concerned with how to guide automatism, and they have carried out various experimental attempts to develop the unconscious, such as inner reflection when in a non-lucid state. They sought new beauty in art, esteeming chance encounters with objects or happenings, as well as states such as surprise, mental turmoil, or madness, as means to rescue the imagination from the tyranny of reason. In their art, they drew on automatist elements through experiments with techniques such as collage [
], frottage [ ], decalcomania [ ], and rayographs [ ]. Breton saw the beauty generated in this way as a marvel that he called “convulsive beauty.” A frequent feature of surrealist expression is dépaysement, in which things are displaced from their usual context [ ]. Rhymes that in reality would not be found together may appear suddenly, or space and time may be distorted, confounding, and unsettling the viewer. The object outside its normal surroundings is not exactly unreal; this is a second reality that exists freely in the subconscious of the surrealist. True freedom, unbound by the rules, restrictions, and restraints that surround the artist, can be found in this second reality [ ].Art and science are seemingly incompatible with each other—but, there is only one thought to bridge the gap between the artistic concept and the scientific phenomenon. Both concepts are keen to meet serendipity to earn originality and creativity.
Biology is the science that seeks to explain the mechanisms and principles of organisms and their environment through careful observation of nature. Microbiology, the study of organisms that are invisible to the naked eye, started in the 17th century when Leeuwenhoek used microscopes that he built himself to show the world for the first time the existence of tiny animals that could not normally be seen [
, ]. Microorganisms are living beings, and the theoretical development of microbiology basically takes place within the system of biology. What, then, would happen if we tried to orient microbiology toward surrealism? Surrealism is the attempt to view reality taken to its limits. Biology starts with close observation of the natural world, but the researcher must not look at nature without life, the object of his or her study. Just as the surrealists did not believe in reality, microbiology denies nature—in this case, reality—to devise and make real environments that could not exist in nature. Present-day advances in refining and synthesis mean that we can create substances or their states that do not exist in nature. Thanks to the advance of technologies for physical devices that can generate and control the movement of different substances, we are now able to create environments that would be unthinkable in nature [ ].The move of microbiology toward surrealism begins with the question of which principles exist in microorganisms when they are exposed to conditions that could not exist on Earth. The field of research that begins with this question is called “surrealistic microbiology.” This is the same as a purely automatic mental phenomenon, and it is a thought process that is far removed from any of the scientific systems built up by our forebears. An environment that could not exist in the natural world can be put together in front of the eyes of the researcher, who can then use a variety of different techniques to observe the state of microorganisms in this environment. The state created by the researcher that would be impossible in the natural world does not exist in nature, but it undoubtedly exists before the eyes of the researcher—it may be regarded as nature taken beyond its limits. One may consider that, just as the surrealists expressed a second reality, surrealistic microbiology sees a second nature. Like nature, this second nature is a phenomenon that occurs on Earth. The human brain is nature, and likewise, the second nature is also nature. Surrealistic microbiology is certainly included within the essential approach of science that seeks to answer the question of what is happening at a particular time. It ensures that the beginning is the true freedom of mind of the researcher, unbound by conventional rules, restrictions, and restraints. The important point here is that those who choose to express themselves through surrealistic microbiology should focus on the application of the new principles discovered through its pursuit. This is because the new principles will gain beauty through their application, becoming a glittering light for future generations. The author proposes microbiology that incorporates the ideas of surrealism—surrealistic microbiology—as a new way of scientific thinking while stating that the new principles must be used for the well-being of humankind, and never as weapons to hurt or kill people; the author therefore announce the birth of surrealistic microbiology.
During passage culture or single colony isolation, an appropriate quantity of bacterial cell suspension is dropped onto nutrient agar and spread across the surface with a streak bar; for researchers who regularly handle bacteria, this is an unremarkable operation that they probably give little thought to. The method of bacterial purification has been followed since it was first devised by R. Koch [
]. If we attempt a physical interpretation of what happens, we can see that sliding friction occurs at the interface between the agar culture medium and the streak bar, so that a friction field is formed. The friction of a hydrogel like agar varies greatly depending on whether the surface is wet or dry: there is less frictional force when the surface of the hydrogel is wet and more when the surface is dry [ ]. The friction of a hydrogel is complex, and its interpretation is not as clear-cut as the friction of a solid. From a physical perspective, the bacterial cells are placed in a sliding friction field at the interface between the agar medium and the streak bar. For the bacteria, this state of being placed in a friction field on a hydrogel is a state that is not found in nature, and it can therefore be taken as being in the second nature. Nano-acicular materials are nano-sized, needle-shaped particles that exist in the natural world or are produced artificially, their existence having no causal relationship with bacterial cells. However, when a nano-acicular material and bacterial cells are brought together in a sliding friction field on a hydrogel, they become able to manifest the physical phenomenon called the Yoshida effect, which has universality [ ].The Yoshida effect is the phenomenon in which bacterial cells and nano-acicular materials in a hydrogel friction field collide with each other, forming fusion bodies called penetrons [
]. A penetron is a bacterial cell pierced by a particle of nano-acicular material. In order for the Yoshida effect to occur, the basic factors are the nano-acicular material and the bacterial cells, and the other essential factors are a hydrogel, a material forming an interface, sliding friction, and a source of energy to provide this friction. Hydrogels include agar, gellan gum, and κ-carrageenan, and a strength of 2.1 N or greater is required. Interface-forming materials include macromolecular substances such as polystyrene, polyethylene, and acrylic nitrile butane diene rubber. Nano-acicular materials include multi-walled carbon nanotubes, γ-maghemite, and α-sepiolite. Energy sources for imparting sliding friction include rotational energy. A normal force of 40 gf/cm2 is suitable for the interface-forming material.A colloidal suspension of bacterial cells and nano-acicular material is placed in the interface between the hydrogel and the interface-forming material, and a sliding friction stimulus is applied. If W is the normal force (gf/cm2) and µ is the coefficient of friction, the frictional force F may be expressed as
F = µW
[
]. The frictional force may be increased by increasing the normal force W, the coefficient of friction µ, or both. When sliding friction is applied with a constant normal force (40 gf/cm2) for a time of 15 s to agar gel with a surface layer of water molecules, the coefficient of friction µ increases from 0.081 to 0.233 with 5% agar gel and from 0.038 to 0.078 with 2% agar gel. The coefficient of friction increases as the water on the surface of the agar gel permeates into the gel. A large coefficient of friction is needed for the Yoshida effect to occur, and when the coefficient of friction reaches its maximum, some unknown force is produced that causes the bacterial cells and the nano-acicular material to collide. The nature of this force, which gives rise to the formation of penetrons, is unclear. It is related not simply to the increase in the coefficient of friction, but rather to the rate of increase. It therefore appears that the unknown force depends on the rate at which the layer of water molecules present on the surface of the hydrogel permeates into the hydrogel. From this, the unknown force F’ in the sliding friction field may be expressed asF’ = (V0-Vs)Ws-2
Where s is the time of application of the sliding friction (s), V0 is the amount of water (ml) in the water molecule layer prior to application of the friction stimulus, Vs is the amount of water (ml) remaining in the water molecule layer after the friction field has been generated for s seconds, and W is the normal force (gf/cm2) applied to the interface-forming material. The unknown force is generated when the water molecules surrounding the bacterial cells and nano-acicular material disappear rapidly.
Penetrons have uniquely fascinating properties. When penetrons are formed by the collision between bacterial cells and nano-acicular material onto which nucleic acid molecules have been adsorbed, transfer of the nucleic acid molecules takes place within the penetrons [
]. Since penetrons are intermediate bodies, they will release daughter cells to try to return to the stable, pre-penetron state if supplied with nutrients and placed in suitable conditions. If a self-replicating plasmid is selected as the nucleic acid molecule, the plasmid will be transmitted to the daughter cells. This property of penetrons has been used to develop technologies for detecting asbestos and for gene transfer to bacteria.Asbestos is a natural fibrous clay mineral, comprised mainly of hydrated silicates. There is great demand for asbestos because it is used in about 3,000 types of industrial goods, including heat-insulating materials for houses and fire-resistant clothing [
]. However, it has been shown to be carcinogenic. As regulations grow more stringent in Europe, the US, and Japan, the importance of asbestos disposal is becoming a major issue [ ]. Over 90% of asbestos is a clay mineral known as chrysotile.It was found that penetrons form when chrysotile and bacterial cells are made to collide in a gel friction field, which led to the development of a method for detecting asbestos using penetrons. If chrysotile is present in a specimen, a penetron will invariably be formed. If the penetron is formed in the presence of a plasmid carrying an antibiotic resistance marker, the penetron will release daughter cells that have become resistant to the antibiotic. In other words, the presence of chrysotile can be estimated in terms of the extent to which the recipient bacteria have incorporated the plasmid and acquired antibiotic resistance [
, ].The specimen must first be crushed in a device such as a pulverizer. The recipient bacterium for penetron formation is E. coli, and plasmids with ampicillin resistance as a marker, such as the pUC series, are relatively easy to handle. The procedure is actually very straightforward. A sample suspension is prepared by suspending the sample to 1 mg/ml and pUC18 to 0.1 µg/ml in pure water. A 2% agar gel containing L broth nutrient source and ampicillin is prepared in 9-cm plates, and 50 µl each of the sample suspension and E. coli culture are dropped onto the surface. A sliding friction stimulus is applied for 30 s using a polystyrene spreader to complete the preparation. The cultures are kept warm for a suitable length of time, and depending on the concentration of chrysotile, ampicillin-resistant colonies appear. The number of ampicillin-resistant colonies that appear is compared to the number that appears when a standard chrysotile suspension (1.0 µg/ml) is used. If the number of ampicillin-resistant colonies to appear from the test sample is greater than the number to appear from the standard chrysotile suspension, the test sample is assumed to contain 0.1% or more chrysotile and is therefore judged to be an asbestos product.
Previous asbestos detection technology involved passing a fixed amount of air through a filter using a suction pump, making the filter transparent, and counting the fibrous particles using a phase-contrast microscope. This method was unable to detect asbestos in soil. The asbestos detection technology based on the Yoshida effect has solved this problem. The asbestos detection technology using penetrons can demonstrate its potential when one wishes to determine whether asbestos is present in soil, rubble, or building materials that may be mixed with other minerals.
Penetrons are formed by causing bacterial cells to collide with nano-acicular material that is coated in (made to adsorb) plasmids. If the penetrons are placed under suitable conditions, they release daughter cells that have acquired the plasmid. This means that a transformation of the bacterial cells has been brought about by the plasmids [
]. In the technology for introducing exogenous genes into bacteria through the mediation of penetrons, α-sepiolite, and chitin nanowhiskers have been confirmed as effective nano-acicular materials. For the hydrogel, agar gel is perfectly adequate for the purpose. Satisfactory results may be obtained by selecting the nano-acicular material to form penetrons according to the species of bacterial cell used and adjusting the strength of the agar gel.The introduction of plasmids into Bacillus subtilis offers the versatility of transformation in a Gram-positive bacterium [
]. The donor plasmid (pHY300PLK), NaCl (to a final concentration of 200 mM), and vegetative cells of the recipient bacteria are suspended in that order in a colloidal suspension of α-sepiolite (1 mg/ml). The resulting colloidal suspension is dripped onto 5% agar gel containing an antibiotic (tetracycline) and LB medium components, and friction stimulation is applied continuously for 60 s with a polystyrene spreader. The gel surface must become dry within 10–20 s; this is critical because if the surface of the gel is still wet after, for example, a minute has passed, no transformants at all will be obtained. After the culture is kept warm for a suitable period, the appearance of antibiotic-resistant colonies can be confirmed.The name “tribos transformation” has been proposed for the technique of gene transfer to bacteria through penetrons, since it is related to frictional force [
]. Unlike conventional methods, tribos transformation does not require the preparation of competent cells or administration of heat shock, and gene transfer and screening are both carried out on the surface of the hydrogel, thus easing some of the complexity [ ]. Plasmid introduction has been confirmed regardless of whether the bacteria are Gram-positive or Gram-negative, and tribos transformation has attracted interest as a novel alternative to conventional gene transfer techniques. Tribos transformation has been shown to be reproducible by several researchers [ - ], indicating the infallibility of the Yoshida effect.When a penetron is formed using nano-acicular material with DNA adsorbed, DNA is exchanged inside the penetron. If the DNA is replaced with antisense nucleic acid, the inhibition of bacterial gene expression can be easily observed from the penetron. The application of the artificial antisense method to bacterial species in which gene introduction has been difficult until now is promising.
The essential factors for the Yoshida effect to occur are a nano-acicular material, a hydrogel, an interface-forming material, and a source of energy to form a friction field. When penetrons are formed by the Yoshida effect, genetic transformation is established if the exchange of nucleic acid materials takes place within the particle and the plasmids or other exogenous molecules are passed on to daughter cells.
A typical nano-acicular material seen in the natural world is chrysotile, which forms crystals in fissures in serpentinite strata [
]. To predict whether the Yoshida effect could occur in the natural world, experiments were carried out with chrysotile as the nano-acicular material, gellan gum as the hydrogel, serpentinite as the interface-forming material, and artificial seismic motion as the energy source to generate the sliding friction field. The frequency of genetic transformations due to plasmids in the daughter cells released by the penetrons was examined [ ]. The recipient cells were Pseudomonas sp. and B. subtilis, and the colloidal suspension containing the donor plasmids and the chrysotile was placed on the surface of the gellan gum, which is a biofilm analog. A serpentine plate was brought into contact with the surface of the gellan gum and subjected to lateral vibrations simulating an earthquake (30–520 gals) to create a friction field. The friction stimulation caused the uptake of the donor plasmid into the recipient cells, promoting transformation to antibiotic resistance. Hard gellan gum promoted plasmid uptake into Pseudomonas sp. and B. subtilis, while there was no uptake with soft gellan gum. With soft gellan gum, permeation of the layer of water molecules was noticeably slow. Uptake of the plasmids by the bacterial cells at the gellan gum interface required the presence of the acicular clay mineral chrysotile together with lateral vibration and the formation of a friction field. The results of the experiment indicate that when biofilms that form in fissures in serpentine are subjected to seismic motion, the Yoshida effect can readily occur with the result that bacteria on the surface of the biofilm become penetrons, and genetic changes due to exogenous genes take place. It has been hypothesized that seismic motion in serpentine strata is a driving force for bacterial evolution in biofilms in serpentine fissures (the earthquake-friction evolution theory).Serpentine layers are not rare on the Earth’s surface and are common in the continental crust. Accumulating evidence showing that microbial genetic diversity is higher in serpentine layers than in other layers would provide strong support for these hypotheses.
Based on a series of observations of the planet Mars, Perron, et al. suggested that the northern plains, which cover almost a third of the surface of the planet, may once have been an ocean [
]. It is believed that peridotite in the uppermost layer of the mantle reacted with water as Mars cooled, transforming it into hydrous minerals such as serpentine, and there are certainly many serpentized peridotites on the surface of the planet [ , ]. From this finding, it may well be assumed that chrysotile is present in fissures in the serpentine.The dust that frequently blows across the surface of Mars in vast storms that engulf the whole planet is from a mineral called maghemite [
]. This mineral is one of the nano-acicular materials that can cause the Yoshida effect.There is a theory that there were continual plate movements for a period during the early stages of the planet’s formation [
], in which case there would have been frequent quakes brought on by the shifting plates. Since there is presently no plate movement on Mars, frequent quakes caused by similar mechanisms to those on Earth are unlikely. Nonetheless, studies of the moon have suggested that gas emissions from the core may be responsible for moonquakes [ ], and it is possible that the same thing occurs on Mars as well. Also, it is thought that massive meteorites rained down in great numbers on the ancient planetary bodies [ ]. The energy from the impact of these massive rocks hitting the surface of the planet would surely be a sufficient source of energy of friction to trigger the Yoshida effect.Mars thus has a nano-acicular material, an interface-forming material, and an energy source such as quakes to apply sliding friction, which are essential factors for the Yoshida effect to occur. The conditions for the effect are therefore in place. Perhaps the Yoshida effect is induced by seismic motion, generating penetrons that are the precursors of genetic transformants; could this be a common principle underlying the evolution of life in the solar system?
When bacterial cells and a nano-acicular material are placed in the friction field of a hydrogel, the two collide to form fusion bodies known as penetrons. Technologies for gene transfer in bacteria and asbestos detection have been deduced from this physical phenomenon. This method, called tribos transformation, is virtually impossible to derive through in-depth observation of nature; it can only be perceived as a possibility with the awareness that the bacteria are placed in a state that could not exist in the natural world. The Yoshida effect is a phenomenon that is mediated through extremely artificial actions, but there is hope that he/she may be able to reach inductive conclusions by attempting to find its significance in the natural world. The research field that places microorganisms in states that could not possibly exist naturally on Earth is called surrealistic microbiology. This paper’s hope is that by creating unique states that would not be found on Earth and setting conditions that go beyond our normal assumptions, it may be able to share, at least to some small extent, a methodology that transcends conventional rules and restrictions.
Breton A. Manifeste du surréalisme. Paris: Ed. Du Sagittaire. 1924.
Adam Z. Alternative modernism: revisiting the 'radical' movement of Dadaism and surrealism. Inter Peer Reviewed/Refereed Multidiscip J. 2022;11:286-289.
Paung Y. Surrealism: Art of Subconscious. 2017.
Fawaz R, Sellier A, Beucler N, Lozouet M, Delmas JM, Desse N, Dagain A. The Origin of Surrealism: Rethinking Apollinaire's Penetrating Brain Injury with Current Knowledge Regarding White Matter Tracts. World Neurosurg. 2023 May;173:44-47. doi: 10.1016/j.wneu.2023.01.121. Epub 2023 Feb 4. PMID: 36739894.
Fer B. Surrealism, myth and psychoanalysis. In: Realism, rationalism, surrealism: Art between the wars. 1993:170-249.
Adibi AA. A brief history of collage. In: Collage: A process in architectural design. 2021:1-5.
Iversen M. Indexical drawing: on frottage. In: A Companion to Contemporary Drawing. 2020:257-270.
Zuena M, Pensabene Buemi L, Nodari L, et al. Portrait of an artist at work: exploring Max Ernst’s surrealist techniques. Herit Sci. 2022;10:139.
Loizos Y. The possibilities of surrealist photography to architectural design and proposition. AIS-Arch Imag Stud. 2020;1:67-75.
Ji H, Park J. The dépaysement art of the new media era incorporating the microscopic world. Digit Creat. 2021;32(2):124-142.
Orlich IA. Surrealism and the feminine element: André Breton’s Nadja and Gellu Naum’s Zenobia. Philologica Jassyensia. 2006;2:213-224.
van Zuylen J. The microscopes of Antoni van Leeuwenhoek. J Microsc. 1981 Mar;121(Pt 3):309-28. doi: 10.1111/j.1365-2818.1981.tb01227.x. PMID: 7012367.
Robertson LA. Antoni van Leeuwenhoek 1723-2023: a review to commemorate Van Leeuwenhoek's death, 300 years ago : For submission to Antonie van Leeuwenhoek journal of microbiology. Antonie Van Leeuwenhoek. 2023 Oct;116(10):919-935. doi: 10.1007/s10482-023-01859-4. Epub 2023 Jul 31. PMID: 37525002; PMCID: PMC10509104.
Deguchi S, Shimoshige H, Tsudome M, Mukai SA, Corkery RW, Ito S, Horikoshi K. Microbial growth at hyperaccelerations up to 403,627 x g. Proc Natl Acad Sci U S A. 2011 May 10;108(19):7997-8002. doi: 10.1073/pnas.1018027108. Epub 2011 Apr 25. PMID: 21518884; PMCID: PMC3093466.
Byrd AL, Segre JA. Infectious disease. Adapting Koch's postulates. Science. 2016 Jan 15;351(6270):224-6. doi: 10.1126/science.aad6753. PMID: 26816362.
Okawara H, Shinomiya K, Nonomura Y. Friction Dynamics on Rough Agar Gel Surfaces. J Oleo Sci. 2019 Sep 4;68(9):873-879. doi: 10.5650/jos.ess19099. Epub 2019 Aug 14. PMID: 31413244.
Yoshida N. Discovery and application of the Yoshida effect: nano-sized acicular materials enable penetration of bacterial cells by sliding friction force. Recent Pat Biotechnol. 2007;1(3):194-201. doi: 10.2174/187220807782330147. PMID: 19075841.
Yoshida N, Sato M. Plasmid uptake by bacteria: a comparison of methods and efficiencies. Appl Microbiol Biotechnol. 2009 Jul;83(5):791-8. doi: 10.1007/s00253-009-2042-4. Epub 2009 May 27. PMID: 19471921.
Popova E, Popov VL. The research works of Coulomb and Amontons and generalized laws of friction. Friction. 2015;3:183-190.
Yoshida N, Ide K. Plasmid DNA is released from nanosized acicular material surface by low molecular weight oligonucleotides: exogenous plasmid acquisition mechanism for penetration intermediates based on the Yoshida effect. Appl Microbiol Biotechnol. 2008 Oct;80(5):813-21. doi: 10.1007/s00253-008-1637-5. Epub 2008 Aug 13. PMID: 18704395.
Virta RL. Asbestos: Geology, mineralogy, mining, and uses. Washington, DC: US Department of the Interior, US Geological Survey; 2002. p. 28.
Wallis SL, Emmett EA, Hardy R, Casper BB, Blanchon DJ, Testa JR, Menges CW, Gonneau C, Jerolmack DJ, Seiphoori A, Steinhorn G, Berry TA. Challenging Global Waste Management - Bioremediation to Detoxify Asbestos. Front Environ Sci. 2020 Mar;8:20. doi: 10.3389/fenvs.2020.00020. Epub 2020 Mar 4. PMID: 33269243; PMCID: PMC7707057.
Yoshida N, Takebe K. Quantitative detection of asbestos fiber in gravelly sand using elastic body-exposure method. J Ind Microbiol Biotechnol. 2006 Oct;33(10):827-33. doi: 10.1007/s10295-006-0125-0. Epub 2006 Apr 25. PMID: 16636778.
Yoshida N, Ikeda T, Yoshida T, Sengoku T, Ogawa K. Chrysotile asbestos fibers mediate transformation of Escherichia coli by exogenous plasmid DNA. FEMS Microbiol Lett. 2001 Feb 20;195(2):133-7. doi: 10.1111/j.1574-6968.2001.tb10510.x. PMID: 11179641.
Yoshida N, Kodama K, Nakata K, Yamashita M, Miwa T. Escherichia coli cells penetrated by chrysotile fibers are transformed to antibiotic resistance by incorporation of exogenous plasmid DNA. Appl Microbiol Biotechnol. 2002 Dec;60(4):461-8. doi: 10.1007/s00253-002-1148-8. Epub 2002 Oct 18. PMID: 12466888.
Green MR, Sambrook J. Easy Transformation of Escherichia coli: Nanoparticle-Mediated Transformation. Cold Spring Harb Protoc. 2019 Dec 2;2019(12). doi: 10.1101/pdb.prot101204. PMID: 31792143.
Tan H, Fu L, Seno M. Optimization of bacterial plasmid transformation using nanomaterials based on the Yoshida effect. Int J Mol Sci. 2010;11(12):4961-72. doi: 10.3390/ijms11124962. Epub 2010 Dec 3. PMID: 21614185; PMCID: PMC3100829.
Wilharm G, Lepka D, Faber F, Hofmann J, Kerrinnes T, Skiebe E. A simple and rapid method of bacterial transformation. J Microbiol Methods. 2010 Feb;80(2):215-6. doi: 10.1016/j.mimet.2009.12.002. PMID: 20004690.
Rodríguez-Beltrán J, Elabed H, Gaddour K, Blázquez J, Rodríguez-Rojas A. Simple DNA transformation in Pseudomonas based on the Yoshida effect. J Microbiol Methods. 2012 May;89(2):95-8. doi: 10.1016/j.mimet.2012.02.013. Epub 2012 Mar 3. PMID: 22405834.
Mincea M, Negrulescu A, Ostafe V. Preparation, modification, and applications of chitin nanowhiskers: a review. Rev Adv Mater Sci. 2012;30:225-242.
Mendes GP, Vieira PS, Lanceros-Méndez S, Kluskens LD, Mota M. Transformation of Escherichia coli JM109 using pUC19 by the Yoshida effect. J Microbiol Methods. 2015 Aug;115:1-5. doi: 10.1016/j.mimet.2015.05.012. Epub 2015 May 9. PMID: 25966644.
Elabed H, Hamza R, Bakhrouf A, Gaddour K. Rapid DNA transformation in Salmonella Typhimurium by the hydrogel exposure method. J Microbiol Methods. 2016 Jul;126:67-71. doi: 10.1016/j.mimet.2016.04.017. Epub 2016 May 3. PMID: 27154729.
Castro-Smirnov FA, Piétrement O, Aranda P, Bertrand JR, Ayache J, Le Cam E, Ruiz-Hitzky E, Lopez BS. Physical interactions between DNA and sepiolite nanofibers, and potential application for DNA transfer into mammalian cells. Sci Rep. 2016 Nov 3;6:36341. doi: 10.1038/srep36341. PMID: 27808269; PMCID: PMC5093858.
Ren J, Karna S, Lee HM, Yoo SM, Na D. Artificial transformation methodologies for improving the efficiency of plasmid DNA transformation and simplifying its use. Appl Microbiol Biotechnol. 2019 Dec;103(23-24):9205-9215. doi: 10.1007/s00253-019-10173-x. Epub 2019 Oct 24. PMID: 31650193.
González-Tortuero E, Rodríguez-Beltrán J, Radek R, Blázquez J, Rodríguez-Rojas A. Clay-induced DNA breaks as a path for genetic diversity, antibiotic resistance, and asbestos carcinogenesis. Sci Rep. 2018 May 31;8(1):8504. doi: 10.1038/s41598-018-26958-5. PMID: 29855603; PMCID: PMC5981458.
Piétrement O, Castro-Smirnov FA, Le Cam E, Aranda P, Ruiz-Hitzky E, Lopez BS. Sepiolite as a New Nanocarrier for DNA Transfer into Mammalian Cells: Proof of Concept, Issues and Perspectives. Chem Rec. 2018 Jul;18(7-8):849-857. doi: 10.1002/tcr.201700078. Epub 2017 Dec 29. PMID: 29286197.
Ren J, Lee H, Yoo SM, Yu MS, Park H, Na D. Combined chemical and physical transformation method with RbCl and sepiolite for the transformation of various bacterial species. J Microbiol Methods. 2017 Apr;135:48-51. doi: 10.1016/j.mimet.2017.02.001. Epub 2017 Feb 7. PMID: 28185866.
Kumari M, Pandey S, Mishra A, Nautiyal CS. Finding a facile way for the bacterial DNA transformation by biosynthesized gold nanoparticles. FEMS Microbiol Lett. 2017 Jul 3;364(12). doi: 10.1093/femsle/fnx081. PMID: 28927194.
Castro-Smirnov FA, Piétrement O, Aranda P, et al. Biotechnological applications of the sepiolite interactions with bacteria: bacterial transformation and DNA extraction. Appl Clay Sci. 2020;191:105613.
Mendes GP, Kluskens LD, Lanceros-Méndez S, Mota M. Magnesium aminoclays as plasmid delivery agents for non-competent Escherichia coli JM109 transformation. Appl Clay Sci. 2021;204:106010.
Mendes GP, Kluskens LD, Mota M, Lanceros-Méndez S, Hatton TA. Spherical and needle-shaped magnetic nanoparticles for friction and magnetic stimulated transformation of microorganisms. Nano-Struct Nano-Objects. 2021;26:100732.
Ragu S, Piétrement O, Lopez BS. Binding of DNA to natural sepiolite: applications in biotechnology and perspectives. Clays Clay Miner. 2021;69:633-640.
Sheridan PO, Odat MA, Scott KP. Establishing genetic manipulation for novel strains of human gut bacteria. Microbiome Res Rep. 2023 Jan 3;2(1):1. doi: 10.20517/mrr.2022.13. PMID: 38059211; PMCID: PMC10696588.
Biddeci G, Spinelli G, Colomba P, Di Blasi F. Halloysite Nanotubes and Sepiolite for Health Applications. Int J Mol Sci. 2023 Mar 2;24(5):4801. doi: 10.3390/ijms24054801. PMID: 36902232; PMCID: PMC10003602.
Enju S, Uehara S, Inoo T. Polygonal serpentine and chrysotile in the Kurosegawa Belt, Kyushu, Japan. Can J Min Pet. 2023;61:145-166.
Somiya Y, Higo N, Yoshida N. Combination of rolling vibration and serpentinite induces the formation of penetration-intermediates. Geomicrobiol J. 2012;29:820-829.
Perron JT, Mitrovica JX, Manga M, Matsuyama I, Richards MA. Evidence for an ancient martian ocean in the topography of deformed shorelines. Nature. 2007 Jun 14;447(7146):840-3. doi: 10.1038/nature05873. PMID: 17568743.
Váci Z, Agee CB, Herd CD, et al. Hydrous olivine alteration on Mars and Earth. Meteorit Planet Sci. 2020;55:1011-1030.
Sánchez-García L, Carrizo D, Jiménez-Gavilán P, Ojeda L, Parro V, Vadillo I. Serpentinization-associated travertines as spatio-temporal archives for lipid biomarkers key for the search for life on Mars. Sci Total Environ. 2024 Feb 20;912:169045. doi: 10.1016/j.scitotenv.2023.169045. Epub 2023 Dec 5. PMID: 38061658.
Jiang Z, Liu Q, Roberts AP, Dekkers MJ, Barrón V, Torrent J, Li S. The magnetic and color reflectance properties of hematite: from Earth to Mars. Rev Geophys. 2022;60
Sleep NH. Martian plate tectonics. J Geophys Res Planets. 1994;99(E3):5639-5655.
Nunn C, Garcia RF, Nakamura Y, et al. Lunar seismology: a data and instrumentation review. Space Sci Rev. 2020;216:89.
Osinski GR, Cockell CS, Pontefract A, Sapers HM. The Role of Meteorite Impacts in the Origin of Life. Astrobiology. 2020 Sep;20(9):1121-1149. doi: 10.1089/ast.2019.2203. Epub 2020 Sep 1. PMID: 32876492; PMCID: PMC7499892.
Yoshida N. A New Physical Phenomenon Discovered When Microbiology Meets Surrealism: The Yoshida Effect has the Power to Fuse Bacteria and Nano-Acicular Materials. IgMin Res. July 31, 2024; 2(7): 687-693. IgMin ID: igmin233; DOI: 10.61927/igmin233; Available at: igmin.link/p233
次のリンクを共有した人は、このコンテンツを読むことができます:
Address Correspondence:
Naoto Yoshida, Department of Biochemistry and Applied Biosciences, University of Miyazaki, 1-1 Gakuen Kibanadai-Nishi, Miyazaki 889-2192, Japan, Email: a04109u@cc.miyazaki-u.ac.jp
How to cite this article:
Yoshida N. A New Physical Phenomenon Discovered When Microbiology Meets Surrealism: The Yoshida Effect has the Power to Fuse Bacteria and Nano-Acicular Materials. IgMin Res. July 31, 2024; 2(7): 687-693. IgMin ID: igmin233; DOI: 10.61927/igmin233; Available at: igmin.link/p233
Copyright: © 2024 Yoshida N This is an open access article distributed under the Creative Commons Attribution License, which permits unrestricted use, distribution, and reproduction in any medium, provided the original work is properly cited.
Breton A. Manifeste du surréalisme. Paris: Ed. Du Sagittaire. 1924.
Adam Z. Alternative modernism: revisiting the 'radical' movement of Dadaism and surrealism. Inter Peer Reviewed/Refereed Multidiscip J. 2022;11:286-289.
Paung Y. Surrealism: Art of Subconscious. 2017.
Fawaz R, Sellier A, Beucler N, Lozouet M, Delmas JM, Desse N, Dagain A. The Origin of Surrealism: Rethinking Apollinaire's Penetrating Brain Injury with Current Knowledge Regarding White Matter Tracts. World Neurosurg. 2023 May;173:44-47. doi: 10.1016/j.wneu.2023.01.121. Epub 2023 Feb 4. PMID: 36739894.
Fer B. Surrealism, myth and psychoanalysis. In: Realism, rationalism, surrealism: Art between the wars. 1993:170-249.
Adibi AA. A brief history of collage. In: Collage: A process in architectural design. 2021:1-5.
Iversen M. Indexical drawing: on frottage. In: A Companion to Contemporary Drawing. 2020:257-270.
Zuena M, Pensabene Buemi L, Nodari L, et al. Portrait of an artist at work: exploring Max Ernst’s surrealist techniques. Herit Sci. 2022;10:139.
Loizos Y. The possibilities of surrealist photography to architectural design and proposition. AIS-Arch Imag Stud. 2020;1:67-75.
Ji H, Park J. The dépaysement art of the new media era incorporating the microscopic world. Digit Creat. 2021;32(2):124-142.
Orlich IA. Surrealism and the feminine element: André Breton’s Nadja and Gellu Naum’s Zenobia. Philologica Jassyensia. 2006;2:213-224.
van Zuylen J. The microscopes of Antoni van Leeuwenhoek. J Microsc. 1981 Mar;121(Pt 3):309-28. doi: 10.1111/j.1365-2818.1981.tb01227.x. PMID: 7012367.
Robertson LA. Antoni van Leeuwenhoek 1723-2023: a review to commemorate Van Leeuwenhoek's death, 300 years ago : For submission to Antonie van Leeuwenhoek journal of microbiology. Antonie Van Leeuwenhoek. 2023 Oct;116(10):919-935. doi: 10.1007/s10482-023-01859-4. Epub 2023 Jul 31. PMID: 37525002; PMCID: PMC10509104.
Deguchi S, Shimoshige H, Tsudome M, Mukai SA, Corkery RW, Ito S, Horikoshi K. Microbial growth at hyperaccelerations up to 403,627 x g. Proc Natl Acad Sci U S A. 2011 May 10;108(19):7997-8002. doi: 10.1073/pnas.1018027108. Epub 2011 Apr 25. PMID: 21518884; PMCID: PMC3093466.
Byrd AL, Segre JA. Infectious disease. Adapting Koch's postulates. Science. 2016 Jan 15;351(6270):224-6. doi: 10.1126/science.aad6753. PMID: 26816362.
Okawara H, Shinomiya K, Nonomura Y. Friction Dynamics on Rough Agar Gel Surfaces. J Oleo Sci. 2019 Sep 4;68(9):873-879. doi: 10.5650/jos.ess19099. Epub 2019 Aug 14. PMID: 31413244.
Yoshida N. Discovery and application of the Yoshida effect: nano-sized acicular materials enable penetration of bacterial cells by sliding friction force. Recent Pat Biotechnol. 2007;1(3):194-201. doi: 10.2174/187220807782330147. PMID: 19075841.
Yoshida N, Sato M. Plasmid uptake by bacteria: a comparison of methods and efficiencies. Appl Microbiol Biotechnol. 2009 Jul;83(5):791-8. doi: 10.1007/s00253-009-2042-4. Epub 2009 May 27. PMID: 19471921.
Popova E, Popov VL. The research works of Coulomb and Amontons and generalized laws of friction. Friction. 2015;3:183-190.
Yoshida N, Ide K. Plasmid DNA is released from nanosized acicular material surface by low molecular weight oligonucleotides: exogenous plasmid acquisition mechanism for penetration intermediates based on the Yoshida effect. Appl Microbiol Biotechnol. 2008 Oct;80(5):813-21. doi: 10.1007/s00253-008-1637-5. Epub 2008 Aug 13. PMID: 18704395.
Virta RL. Asbestos: Geology, mineralogy, mining, and uses. Washington, DC: US Department of the Interior, US Geological Survey; 2002. p. 28.
Wallis SL, Emmett EA, Hardy R, Casper BB, Blanchon DJ, Testa JR, Menges CW, Gonneau C, Jerolmack DJ, Seiphoori A, Steinhorn G, Berry TA. Challenging Global Waste Management - Bioremediation to Detoxify Asbestos. Front Environ Sci. 2020 Mar;8:20. doi: 10.3389/fenvs.2020.00020. Epub 2020 Mar 4. PMID: 33269243; PMCID: PMC7707057.
Yoshida N, Takebe K. Quantitative detection of asbestos fiber in gravelly sand using elastic body-exposure method. J Ind Microbiol Biotechnol. 2006 Oct;33(10):827-33. doi: 10.1007/s10295-006-0125-0. Epub 2006 Apr 25. PMID: 16636778.
Yoshida N, Ikeda T, Yoshida T, Sengoku T, Ogawa K. Chrysotile asbestos fibers mediate transformation of Escherichia coli by exogenous plasmid DNA. FEMS Microbiol Lett. 2001 Feb 20;195(2):133-7. doi: 10.1111/j.1574-6968.2001.tb10510.x. PMID: 11179641.
Yoshida N, Kodama K, Nakata K, Yamashita M, Miwa T. Escherichia coli cells penetrated by chrysotile fibers are transformed to antibiotic resistance by incorporation of exogenous plasmid DNA. Appl Microbiol Biotechnol. 2002 Dec;60(4):461-8. doi: 10.1007/s00253-002-1148-8. Epub 2002 Oct 18. PMID: 12466888.
Green MR, Sambrook J. Easy Transformation of Escherichia coli: Nanoparticle-Mediated Transformation. Cold Spring Harb Protoc. 2019 Dec 2;2019(12). doi: 10.1101/pdb.prot101204. PMID: 31792143.
Tan H, Fu L, Seno M. Optimization of bacterial plasmid transformation using nanomaterials based on the Yoshida effect. Int J Mol Sci. 2010;11(12):4961-72. doi: 10.3390/ijms11124962. Epub 2010 Dec 3. PMID: 21614185; PMCID: PMC3100829.
Wilharm G, Lepka D, Faber F, Hofmann J, Kerrinnes T, Skiebe E. A simple and rapid method of bacterial transformation. J Microbiol Methods. 2010 Feb;80(2):215-6. doi: 10.1016/j.mimet.2009.12.002. PMID: 20004690.
Rodríguez-Beltrán J, Elabed H, Gaddour K, Blázquez J, Rodríguez-Rojas A. Simple DNA transformation in Pseudomonas based on the Yoshida effect. J Microbiol Methods. 2012 May;89(2):95-8. doi: 10.1016/j.mimet.2012.02.013. Epub 2012 Mar 3. PMID: 22405834.
Mincea M, Negrulescu A, Ostafe V. Preparation, modification, and applications of chitin nanowhiskers: a review. Rev Adv Mater Sci. 2012;30:225-242.
Mendes GP, Vieira PS, Lanceros-Méndez S, Kluskens LD, Mota M. Transformation of Escherichia coli JM109 using pUC19 by the Yoshida effect. J Microbiol Methods. 2015 Aug;115:1-5. doi: 10.1016/j.mimet.2015.05.012. Epub 2015 May 9. PMID: 25966644.
Elabed H, Hamza R, Bakhrouf A, Gaddour K. Rapid DNA transformation in Salmonella Typhimurium by the hydrogel exposure method. J Microbiol Methods. 2016 Jul;126:67-71. doi: 10.1016/j.mimet.2016.04.017. Epub 2016 May 3. PMID: 27154729.
Castro-Smirnov FA, Piétrement O, Aranda P, Bertrand JR, Ayache J, Le Cam E, Ruiz-Hitzky E, Lopez BS. Physical interactions between DNA and sepiolite nanofibers, and potential application for DNA transfer into mammalian cells. Sci Rep. 2016 Nov 3;6:36341. doi: 10.1038/srep36341. PMID: 27808269; PMCID: PMC5093858.
Ren J, Karna S, Lee HM, Yoo SM, Na D. Artificial transformation methodologies for improving the efficiency of plasmid DNA transformation and simplifying its use. Appl Microbiol Biotechnol. 2019 Dec;103(23-24):9205-9215. doi: 10.1007/s00253-019-10173-x. Epub 2019 Oct 24. PMID: 31650193.
González-Tortuero E, Rodríguez-Beltrán J, Radek R, Blázquez J, Rodríguez-Rojas A. Clay-induced DNA breaks as a path for genetic diversity, antibiotic resistance, and asbestos carcinogenesis. Sci Rep. 2018 May 31;8(1):8504. doi: 10.1038/s41598-018-26958-5. PMID: 29855603; PMCID: PMC5981458.
Piétrement O, Castro-Smirnov FA, Le Cam E, Aranda P, Ruiz-Hitzky E, Lopez BS. Sepiolite as a New Nanocarrier for DNA Transfer into Mammalian Cells: Proof of Concept, Issues and Perspectives. Chem Rec. 2018 Jul;18(7-8):849-857. doi: 10.1002/tcr.201700078. Epub 2017 Dec 29. PMID: 29286197.
Ren J, Lee H, Yoo SM, Yu MS, Park H, Na D. Combined chemical and physical transformation method with RbCl and sepiolite for the transformation of various bacterial species. J Microbiol Methods. 2017 Apr;135:48-51. doi: 10.1016/j.mimet.2017.02.001. Epub 2017 Feb 7. PMID: 28185866.
Kumari M, Pandey S, Mishra A, Nautiyal CS. Finding a facile way for the bacterial DNA transformation by biosynthesized gold nanoparticles. FEMS Microbiol Lett. 2017 Jul 3;364(12). doi: 10.1093/femsle/fnx081. PMID: 28927194.
Castro-Smirnov FA, Piétrement O, Aranda P, et al. Biotechnological applications of the sepiolite interactions with bacteria: bacterial transformation and DNA extraction. Appl Clay Sci. 2020;191:105613.
Mendes GP, Kluskens LD, Lanceros-Méndez S, Mota M. Magnesium aminoclays as plasmid delivery agents for non-competent Escherichia coli JM109 transformation. Appl Clay Sci. 2021;204:106010.
Mendes GP, Kluskens LD, Mota M, Lanceros-Méndez S, Hatton TA. Spherical and needle-shaped magnetic nanoparticles for friction and magnetic stimulated transformation of microorganisms. Nano-Struct Nano-Objects. 2021;26:100732.
Ragu S, Piétrement O, Lopez BS. Binding of DNA to natural sepiolite: applications in biotechnology and perspectives. Clays Clay Miner. 2021;69:633-640.
Sheridan PO, Odat MA, Scott KP. Establishing genetic manipulation for novel strains of human gut bacteria. Microbiome Res Rep. 2023 Jan 3;2(1):1. doi: 10.20517/mrr.2022.13. PMID: 38059211; PMCID: PMC10696588.
Biddeci G, Spinelli G, Colomba P, Di Blasi F. Halloysite Nanotubes and Sepiolite for Health Applications. Int J Mol Sci. 2023 Mar 2;24(5):4801. doi: 10.3390/ijms24054801. PMID: 36902232; PMCID: PMC10003602.
Enju S, Uehara S, Inoo T. Polygonal serpentine and chrysotile in the Kurosegawa Belt, Kyushu, Japan. Can J Min Pet. 2023;61:145-166.
Somiya Y, Higo N, Yoshida N. Combination of rolling vibration and serpentinite induces the formation of penetration-intermediates. Geomicrobiol J. 2012;29:820-829.
Perron JT, Mitrovica JX, Manga M, Matsuyama I, Richards MA. Evidence for an ancient martian ocean in the topography of deformed shorelines. Nature. 2007 Jun 14;447(7146):840-3. doi: 10.1038/nature05873. PMID: 17568743.
Váci Z, Agee CB, Herd CD, et al. Hydrous olivine alteration on Mars and Earth. Meteorit Planet Sci. 2020;55:1011-1030.
Sánchez-García L, Carrizo D, Jiménez-Gavilán P, Ojeda L, Parro V, Vadillo I. Serpentinization-associated travertines as spatio-temporal archives for lipid biomarkers key for the search for life on Mars. Sci Total Environ. 2024 Feb 20;912:169045. doi: 10.1016/j.scitotenv.2023.169045. Epub 2023 Dec 5. PMID: 38061658.
Jiang Z, Liu Q, Roberts AP, Dekkers MJ, Barrón V, Torrent J, Li S. The magnetic and color reflectance properties of hematite: from Earth to Mars. Rev Geophys. 2022;60
Sleep NH. Martian plate tectonics. J Geophys Res Planets. 1994;99(E3):5639-5655.
Nunn C, Garcia RF, Nakamura Y, et al. Lunar seismology: a data and instrumentation review. Space Sci Rev. 2020;216:89.
Osinski GR, Cockell CS, Pontefract A, Sapers HM. The Role of Meteorite Impacts in the Origin of Life. Astrobiology. 2020 Sep;20(9):1121-1149. doi: 10.1089/ast.2019.2203. Epub 2020 Sep 1. PMID: 32876492; PMCID: PMC7499892.