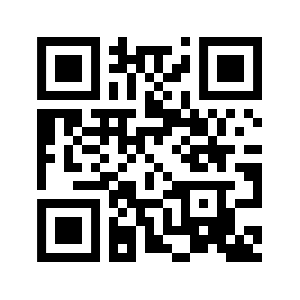
Fibrin Contributes to an Improvement of an in vitro Wound Repair Model using Fibroblast-populated Collagen Lattices
Dermatology Pathology受け取った 19 Feb 2024 受け入れられた 23 Mar 2024 オンラインで公開された 25 Mar 2024
Focusing on Biology, Medicine and Engineering ISSN: 2995-8067 | Quick Google Scholar
Next Full Text
Breast Cancer: The Road to a Personalized Prevention
受け取った 19 Feb 2024 受け入れられた 23 Mar 2024 オンラインで公開された 25 Mar 2024
Incisional acute wounds of the skin are characterized by a rapid biomechanical response by stromal cell contraction that joins the wound lips through the fibrin cloth. In this work, we have performed an in vitro model using Fibroblast-Populated Collagen Lattices (FPCLs) that partially mimic that physiological process. Injured FPCLs under relaxed or stressed conditions were evaluated over time, and cross-sections of the lattices were stained with picrosirius red. Wounds filled with fibrin in relaxed FPCLs were closed earlier than controls, the fibrillar pattern of the collagen lattice was different between the wound and the edges of the lattice. On the other hand, stressed FPCLs did not close wounds, even those filled with fibrin, because the tension generated from the lattice borders maintained high tension towards the wound. Controls or fibrin-treated stressed FPCLs, showed high tension in the wound matrix, characterized by the high packing of collagen observed like yellow-red birefringent fibers when stained by picrosirius red. Despite wounds that remain open, fibrin-treated FPCLs exhibited less wound area than controls. With this work, we have demonstrated that FPCL models can be used to study wound closure, mainly when they are improved with other elements of the wound environment that allow us to analyze the biological process.
Tissue tension in an incisional wound of integumentary organs, such as the skin, immediately spreads out the tissues due to the contraction derived from the surroundings, creating a hollow. Mesenchymal cells attached to the extracellular matrix (ECM) produce mechanical and metabolic changes in tissue architecture [
], while matrix proteins, such as fibrinogen still soluble in the blood, finally polymerize and fill the damage by linking the injury borders [ ]. Stress at the edges of the wound is permanent, and the provisional fibrin matrix is turnover with a transition ECM by stromal cells [ ]. This new ECM is characterized by the presence of collagens in the wound, which favors mainly bidirectional contraction until damage is practically limited to the transition zone [ ], where epithelialization and reconnection of the wound margins become relatively fast. In this simplistic way to describe the wound repair process, tridimensional models of wound healing have been developed, trying to understand how fibroblasts populate the newly formed ECM in the wound bed and how ECM turnover becomes a critical factor [ ].From the different models of in vitro wound healing [
], the fibroblast-populated collagen lattices-based assays have been extensively explored in order to isolate two elements that contribute critically to wound repair, fibroblasts, and collagen [ ]. Mostly, authors perform excisional injuries to the lattices to evaluate fibroblast repopulation of the damage [ ], where it has been possible to know the mechanobiology of fibroblast-collagen [ ]. FPCL-based wound healing models can involve a mechanically relaxed collagenous matrix (free-floating), a stressed matrix, or stressed-relaxed, where the matrix is attached for a while and later released for free-floating [ ]. Each model generates different information because the biomechanical environment is quite distinct, however, the three models have provided information regarding traction forces that stimulate fibroblasts to reorganize collagen fibers. For example, relaxed FPCLs deposit collagen in the direction in which cells spread; nevertheless, collagen turnover in stressed FPCLs is not a key factor for contraction, suggesting that matrix rearrangement by fibroblasts is the main mechanism for contraction [ ]. However, the presence of fibrin in the relaxed FPCLs favors lattice stiffness, decreases contraction, and promotes fibrinolytic activity. Through culture time, collagen-fibrin FPCLs, clear fibrin deposits, ultimately look like fibrin-free FPCLs, indicating that the protein plays an important role in early wound biomechanics [ ]. Based on the above data, in this work, we propose two models for in vitro wound repair; both using FPCLs under relaxed or stressed matrix conditions, with the novelty that the ‘wound’ was incisional and filled with fibrin, in order to mimic the early cloth of the wound, and where the changes were evaluated by histomorphology and morphometry.Immortalized fibroblasts hTERT-BJ1 were cultured with Dulbecco’s Modified Eagle’s Medium (Gibco, Life Technologies. NY, USA) supplemented with 10% fetal bovine serum (Gibco), 2 mM L-glutamine (Gibco), 100 U/ml penicillin, and 100 μg/ml streptomycin (Gibco). Cultures were maintained at 37 °C and 5% CO2, and cells were used to prepare fibroblast-populated collagen lattices (FPCLs) when they reached 70% - 80% confluence. Culture medium (1.5×) and type I collagen (DSM Branch, Pentapharm, Aesch, Switzerland), previously dialyzed versus 0.05 M acetic acid, were mixed in an ice bath to reach a final concentration of 2.0 mg/ml and 1 × 105 cells/ml and 2.5 mg/ml and 4 × 104 cells/ml for relaxed and stressed FPCL, respectively. Two milliliters of the mixture were dispersed in 24-well flat culture plates (191mm2, Costar, Corning, NY, USA] and the lattices were formed by spontaneous polymerization at 37 °C, 5% CO2. Only for relaxed FPCLs, the matrix was released from the borders of each well after the first hour of culture using a sterile needle [
].To mimic incisional wounds under relaxed or stressed matrix conditions, incisional wounds were performed in the FPCLs. For relaxed FPCLs, cultures were left to contract for 3 days. Then, they and stressed cultures 1 day underwent a 50% thick guillotine-style cutting (2 and 5 mm, respectively) in the middle of every lattice with a sterile stainless-steel knife (Figure 1); only for relaxed FPCL contracted lattices were held with a sterilized plastic straw, in order to fix it and allow the clean cut (Figure 1a). For the fibrin-treated groups, wounds were filled with 30 µl of a soluble fibrin matrix (Hemostatic adhesive Tisseel, Baxter. IL, USA) immediately after cut. After fibrin polymerization cultures were incubated together with controls, which did not receive any treatment. All lattices were incubated and duplicates of two independent experiments were evaluated for 10 days. Wounded lattices without cells were used as technical controls.
Figure 1: Devices to create wounds on FPCLs. Standardization of wounds was achieved through a sterilized stainless-steel knife coupled with Kelly forceps. For relaxed FPCL it was necessary to hold the lattice by introducing it into a plastic straw with a slit that would allow the knife to fit exactly 2 mm (a). For stressed FPCLs, the fit distance of the knife was 5 mm measured from the tip of Kelly forceps to the knife edge, in order to reach the exact depth into the de lattice when the tip of the forceps had touched the surface of the FPCLs (b). Created with BioRender.com.
The images of the area of each wound were acquired and measured daily during the incubation period with a Gel-Doc photodocumentation system (Bio-Rad. CA, USA) and Quantity One 4.6.3 software (Bio-Rad).
Both relaxed and stressed lattices were fixed for 1 h, at 4 °C, using 2 ml of 4% paraformaldehyde solution. The FPCLs of the stressed cultures were detached from the well walls and left for an additional 1 h. The relaxed and stressed FPCLs were then placed in polypropylene tubes with 10 ml of fresh 4% paraformaldehyde solution overnight at 4 °C. Lattices were dehydrated using graduated ethanol solutions (50%, 70%, 80%, 96%, and 100%) for 30 min each and were embedded in paraffin. Deparaffinized cross sections of 5 μm thick were stained according to the Picro Sirius Red Kit procedure (AB150681, Abcam Inc., MA, USA), dehydrated, and mounted with Entellan™ (Merck KGaA, Darmstadt, Germany). Photomicrographs that show the collagen fibre pattern of the lattices were acquired at 100 × magnification under an Axio Imager Z.1 microscope (Carl Zeiss, Göttingen, Germany) fitted with a high-speed camera (AxioCam; Carl Zeiss) using the ZEN lite software V. 3.0 (Carl Zeiss).
The wound size data in stressed FPLCs represent duplicates of two independent experiments (n = 4). Two-way ANOVA and Sidak’s multiple comparison tests were performed with GraphPad Prism 8 (GraphPad Software. MA, USA); p values ≤ 0.05 were considered significant.
In this work, we explore two models for incisional wound repair, relaxed and stressed FPCLs. With the first, we have explored how the multidirectional contraction of a relaxed collagen matrix embedded with fibroblasts contributed to wound closure, even when the damage to the lattice was or was not filled with polymerizing fibrin. Although macroscopically both groups closed the wound after 10 days (Figure 2a); histologically, the control cultures still showed the wound compared to the fibrin-treated cultures (Figure 2b). After 1 day of damage, in fibrin-treated cultures, a violet-blue clot was observed that covered the area of the wound and beyond (Figure 2b), the birefringent collagen fibers (green) showed an altered organization pattern close to the wound, compared to the uninjured area (the area away from the damage). Perpendicular yellow-orange fibers were found inside and in the frame of the incision, while in the uninjured area, the fibers were observed randomly distributed. These various birefringent colors reflect differences in the packing of the fibers during the repair process. Orange-yellow fibers were still visible in the wound closure area of the control cultures even after 10 days, whereas the effect was only observed after 1 day post-wound in cultures treated with fibrin. On days 5 and 10, the fibrin fiber pattern of the wound gradually vanished and the green and low-packed collagen fibers dispersed throughout the network similarly to the uninjured area. Differences in relaxed FPCL between controls and fibrin-treated cultures showed a wound healing delay of 10 days, at least by histological analysis.
Figure 2: Relaxed FPCLs. a) Pictures of representative relaxed FPCL of control and fibrin treated at different time points, 1, 5, and 10 days. b) Photomicrographs of cross sections of relaxed FPCLs stained with picrosirius red. The bar represents 200 μm.
The second model we performed was the same kind of wound, but in completely stressed FPCLs, that is, the matrix remained attached to the culture well at the bottom and the borders during the experiment. As in the previous model, contraction was multidirectional, but in this case, stress was much higher and produced a progressively opening wound (Figures 3,4). Despite neither control nor fibrin-treated cultures repairing the wound during the 10-day period assay, clearly, fibrin contributed to slower wound opening (Figure 3a,3b), where fiber packing was more evident in fibrin-treated wounds. At the end of the assay, on day 10, the fibrin-treated cultures did not show a depth injury characterized by highly dense packed collagen fibers as seen in the control cultures (yellow-orange fibers, Figure 3b), although the wound remained open.
Figure 3: Stressed FPCLs. a) Pictures of representative stressed FPCL of control and fibrin treated at different time points, 1, 5, and 10 days. b) Photomicrographs of cross sections of stressed FPCLs stained with picrosirius red. The bar represents 500 μm.
Figure 4: The wound size was measured for stressed FPLCs during culture time. The stressed FPCLs treated with fibrin and control were measured for 10 days and data was plotted. Two-way ANOVA and Sidak’s multiple comparison tests were performed on the data (n = 4). *p = 0.0002, **p ≤ 0.0001. The dashed line indicates the wound size in the technical controls (lattices without cells and without fibrin).
Despite the hundreds of publications on FPCLs used to model wound repair, only one of them has performed injuries with the aim of mimicking an incisional wound. A full-thickness wound was performed that divides the lattice into two portions, and both sections were joined last [
]. Data derived from the work demonstrated that FPCL could be a close model for wound repair, since cell migration and differentiation, as well as synthesis of ECM components, resembles the remodeling process observed during wound healing of the skin, but not regeneration, because the architecture of the repaired lattices looked like a scar [ ]. However, incisional skin wounds involve a section that spreads, such as the lips, while the bottom remains joined; independently of whether the injury is partial or full thickness. So, in this work, we have performed a wound model that resembles the mechanics of incisional damage of the skin, including a cloth, but in this case, we have explored lattice response when they have been relaxed (free-floating) or stressed (attached) because we wanted to know qualitatively the wound and the surrounding tension. It was evident that fibrin contributed to the repair process at a low-stress level; however, when matrix tension was high, the fibroblast traction forces [ ] were able to partially detach collagen-fibrin interactions and spread the edges of the wound over time. Beyond the mechanical information provided by this model, it also involved the cloth processing observed during skin wound repair [ ]. Picrosirius red stained lattices, mainly those of relaxed FPCLs, showed how fibrin vanished over time, leaving less stress in collagen fibers. On day 1 after injury, both fibrin-treated and control cultures showed fibers with a yellow-orange birefringence pattern with a preferential orientation perpendicular to the incision, while in the area beyond the wound, fibers showed a green pattern with a random orientation. This agrees with previous research published by Simon DD et al., who found a marked green birefringence due to collagen fibers randomly oriented in the center and beyond the unwounded relaxed FPCLs [ ]. After 5 days of contraction, near the lattice borders, the fibers showed a preferential alignment parallel to the edge, characterized by a red birefringence, and were associated with more compact and/or thicker fibers [ ]. In the relaxed FPCL wound model presented here, the wound edges were closed, promoting a repair zone with yellow-orange birefringent fibers, suggesting changes in collagen organization in the wound area due to cell spreading and the development of tension forces during wound contraction [ ]. FPCLs as a wound repair model have many limitations [ ] because they are a monocellular culture; fibroblasts, involving a single fibrous protein; type I collagen. However, in this work, we demonstrate that fibroblasts indeed promote matrix contraction that closes the wound, mainly when the damage had been filled with fibrin (mimicking a cloth), demonstrating, at least in part, the transitory role of fibrin during early wound repair when cells contract and turnover the combined matrix [ ].Partial thickness wounds performed in FPCL resemble some mechanic properties of dermal incisional wounds. Fibrin addition to the injury contributes to wound edge closing in free-floating FPCL, favoring matrix repair. Excessive tension in stressed FPCL alters wound closure, where fibrin delays the process. In summary, the injury model in FPCL can work to assess the biomechanical characteristics of the wound repair process, where the addition of every single component of the whole tissue could contribute to understanding pathophysiological conditions during natural wound repair.
Data presented here are Aldo Montes de Oca-Delgado’s work to obtain his Bachelor’s Degree in Biomedical Systems Engineering at the Mechanical and Industrial Engineering Division and of the Division of Electrical Engineering of the Universidad Nacional Autónoma de México. Language revision was performed using Writefull AI.
Kimura S, Tsuji T. Mechanical and Immunological Regulation in Wound Healing and Skin Reconstruction. Int J Mol Sci. 2021 May 22;22(11):5474. doi: 10.3390/ijms22115474. PMID: 34067386; PMCID: PMC8197020.
Reinke JM, Sorg H. Wound repair and regeneration. Eur Surg Res. 2012;49(1):35-43. doi: 10.1159/000339613. Epub 2012 Jul 11. PMID: 22797712.
Bainbridge P. Wound healing and the role of fibroblasts. J Wound Care. 2013 Aug;22(8):407-8, 410-12. doi: 10.12968/jowc.2013.22.8.407. PMID: 23924840.
Ehrlich HP, Hunt TK. Collagen Organization Critical Role in Wound Contraction. Adv Wound Care (New Rochelle). 2012 Feb;1(1):3-9. doi: 10.1089/wound.2011.0311. PMID: 24527271; PMCID: PMC3839005.
Ud-Din S, Bayat A. Non-animal models of wound healing in cutaneous repair: In silico, in vitro, ex vivo, and in vivo models of wounds and scars in human skin. Wound Repair Regen. 2017 Apr;25(2):164-176. doi: 10.1111/wrr.12513. Epub 2017 Feb 20. PMID: 28120405.
Menon SN, Flegg JA. Mathematical Modeling Can Advance Wound Healing Research. Adv Wound Care (New Rochelle). 2021 Jun;10(6):328-344. doi: 10.1089/wound.2019.1132. Epub 2020 Sep 11. PMID: 32634070; PMCID: PMC8082733.
Wolf K, Alexander S, Schacht V, Coussens LM, von Andrian UH, van Rheenen J, Deryugina E, Friedl P. Collagen-based cell migration models in vitro and in vivo. Semin Cell Dev Biol. 2009 Oct;20(8):931-41. doi: 10.1016/j.semcdb.2009.08.005. Epub 2009 Aug 12. PMID: 19682592; PMCID: PMC4021709.
al-Khateeb T, Stephens P, Shepherd JP, Thomas DW. An investigation of preferential fibroblast wound repopulation using a novel in vitro wound model. J Periodontol. 1997 Nov;68(11):1063-9. doi: 10.1902/jop.1997.68.11.1063. PMID: 9407398.
Dallon JC, Ehrlich HP. A review of fibroblast-populated collagen lattices. Wound Repair Regen. 2008 Jul-Aug;16(4):472-9. doi: 10.1111/j.1524-475X.2008.00392.x. PMID: 18638264.
Howard JC, Varallo VM, Ross DC, Roth JH, Faber KJ, Alman B, Gan BS. Elevated levels of beta-catenin and fibronectin in three-dimensional collagen cultures of Dupuytren's disease cells are regulated by tension in vitro. BMC Musculoskelet Disord. 2003 Jul 16;4:16. doi: 10.1186/1471-2474-4-16. PMID: 12866952; PMCID: PMC183833.
Chopin-Doroteo M, Salgado-Curiel RM, Pérez-González J, Marín-Santibáñez BM, Krötzsch E. Fibroblast populated collagen lattices exhibit opposite biophysical conditions by fibrin or hyaluronic acid supplementation. J Mech Behav Biomed Mater. 2018 Jun;82:310-319. doi: 10.1016/j.jmbbm.2018.03.042. Epub 2018 Mar 31. PMID: 29653380.
Lombardi B, Casale C, Imparato G, Urciuolo F, Netti PA. Spatiotemporal Evolution of the Wound Repairing Process in a 3D Human Dermis Equivalent. Adv Healthc Mater. 2017 Jul;6(13). doi: 10.1002/adhm.201601422. Epub 2017 Apr 13. PMID: 28407433.
Simon DD, Horgan CO, Humphrey JD. Mechanical restrictions on biological responses by adherent cells within collagen gels. J Mech Behav Biomed Mater. 2012 Oct;14:216-26. doi: 10.1016/j.jmbbm.2012.05.009. Epub 2012 May 22. PMID: 23022259; PMCID: PMC3516288.
Geer DJ, Swartz DD, Andreadis ST. Fibrin promotes migration in a three-dimensional in vitro model of wound regeneration. Tissue Eng. 2002 Oct;8(5):787-98. doi: 10.1089/10763270260424141. PMID: 12459057.
Costa KD, Lee EJ, Holmes JW. Creating alignment and anisotropy in engineered heart tissue: role of boundary conditions in a model three-dimensional culture system. Tissue Eng. 2003 Aug;9(4):567-77. doi: 10.1089/107632703768247278. PMID: 13678436.
Kuhn MA, Smith PD, Hill DP, Ko F, Meltzer DD, Vande Berg JS, Robson MC. In vitro fibroblast populated collagen lattices are not good models of in vivo clinical wound healing. Wound Repair Regen. 2000 Jul-Aug;8(4):270-6. doi: 10.1046/j.1524-475x.2000.00270.x. PMID: 11013018.
Chopin-Doroteo M, Oca-Delgado AMD, Salgado RO, Krötzsch E. Fibrin Contributes to an Improvement of an in vitro Wound Repair Model using Fibroblast-populated Collagen Lattices. IgMin Res. 25 Mar, 2024; 2(3): 159-162. IgMin ID: igmin159; DOI:10.61927/igmin159; Available at: igmin.link/p159
次のリンクを共有した人は、このコンテンツを読むことができます:
1Laboratory of Connective Tissue, National Center for Research and Care of Burns, “Luis Guillermo Ibarra Ibarra” National Rehabilitation Institute, Mexico City, Mexico
2Faculty of Engineering, Division of Mechanical and Industrial Engineering and the Division of Electrical Engineering, National Autonomous University of Mexico, Mexico City, Mexico
Address Correspondence:
Edgar Krötzsch, Ph.D., Laboratory of Connective Tissue, National Burn Research and Care Center, National Rehabilitation Institute “Luis Guillermo Ibarra Ibarra”, Mexico-Xochimilco Road 289, Guadalupe Sand Colony, Mexico City, Mexico, Email: [email protected]
How to cite this article:
Chopin-Doroteo M, Oca-Delgado AMD, Salgado RO, Krötzsch E. Fibrin Contributes to an Improvement of an in vitro Wound Repair Model using Fibroblast-populated Collagen Lattices. IgMin Res. 25 Mar, 2024; 2(3): 159-162. IgMin ID: igmin159; DOI:10.61927/igmin159; Available at: igmin.link/p159
Copyright: © 2024 Chopin-Doroteo M, et al This is an open access article distributed under the Creative Commons Attribution License, which permits unrestricted use, distribution, and reproduction in any medium, provided the original work is properly cited.
Kimura S, Tsuji T. Mechanical and Immunological Regulation in Wound Healing and Skin Reconstruction. Int J Mol Sci. 2021 May 22;22(11):5474. doi: 10.3390/ijms22115474. PMID: 34067386; PMCID: PMC8197020.
Reinke JM, Sorg H. Wound repair and regeneration. Eur Surg Res. 2012;49(1):35-43. doi: 10.1159/000339613. Epub 2012 Jul 11. PMID: 22797712.
Bainbridge P. Wound healing and the role of fibroblasts. J Wound Care. 2013 Aug;22(8):407-8, 410-12. doi: 10.12968/jowc.2013.22.8.407. PMID: 23924840.
Ehrlich HP, Hunt TK. Collagen Organization Critical Role in Wound Contraction. Adv Wound Care (New Rochelle). 2012 Feb;1(1):3-9. doi: 10.1089/wound.2011.0311. PMID: 24527271; PMCID: PMC3839005.
Ud-Din S, Bayat A. Non-animal models of wound healing in cutaneous repair: In silico, in vitro, ex vivo, and in vivo models of wounds and scars in human skin. Wound Repair Regen. 2017 Apr;25(2):164-176. doi: 10.1111/wrr.12513. Epub 2017 Feb 20. PMID: 28120405.
Menon SN, Flegg JA. Mathematical Modeling Can Advance Wound Healing Research. Adv Wound Care (New Rochelle). 2021 Jun;10(6):328-344. doi: 10.1089/wound.2019.1132. Epub 2020 Sep 11. PMID: 32634070; PMCID: PMC8082733.
Wolf K, Alexander S, Schacht V, Coussens LM, von Andrian UH, van Rheenen J, Deryugina E, Friedl P. Collagen-based cell migration models in vitro and in vivo. Semin Cell Dev Biol. 2009 Oct;20(8):931-41. doi: 10.1016/j.semcdb.2009.08.005. Epub 2009 Aug 12. PMID: 19682592; PMCID: PMC4021709.
al-Khateeb T, Stephens P, Shepherd JP, Thomas DW. An investigation of preferential fibroblast wound repopulation using a novel in vitro wound model. J Periodontol. 1997 Nov;68(11):1063-9. doi: 10.1902/jop.1997.68.11.1063. PMID: 9407398.
Dallon JC, Ehrlich HP. A review of fibroblast-populated collagen lattices. Wound Repair Regen. 2008 Jul-Aug;16(4):472-9. doi: 10.1111/j.1524-475X.2008.00392.x. PMID: 18638264.
Howard JC, Varallo VM, Ross DC, Roth JH, Faber KJ, Alman B, Gan BS. Elevated levels of beta-catenin and fibronectin in three-dimensional collagen cultures of Dupuytren's disease cells are regulated by tension in vitro. BMC Musculoskelet Disord. 2003 Jul 16;4:16. doi: 10.1186/1471-2474-4-16. PMID: 12866952; PMCID: PMC183833.
Chopin-Doroteo M, Salgado-Curiel RM, Pérez-González J, Marín-Santibáñez BM, Krötzsch E. Fibroblast populated collagen lattices exhibit opposite biophysical conditions by fibrin or hyaluronic acid supplementation. J Mech Behav Biomed Mater. 2018 Jun;82:310-319. doi: 10.1016/j.jmbbm.2018.03.042. Epub 2018 Mar 31. PMID: 29653380.
Lombardi B, Casale C, Imparato G, Urciuolo F, Netti PA. Spatiotemporal Evolution of the Wound Repairing Process in a 3D Human Dermis Equivalent. Adv Healthc Mater. 2017 Jul;6(13). doi: 10.1002/adhm.201601422. Epub 2017 Apr 13. PMID: 28407433.
Simon DD, Horgan CO, Humphrey JD. Mechanical restrictions on biological responses by adherent cells within collagen gels. J Mech Behav Biomed Mater. 2012 Oct;14:216-26. doi: 10.1016/j.jmbbm.2012.05.009. Epub 2012 May 22. PMID: 23022259; PMCID: PMC3516288.
Geer DJ, Swartz DD, Andreadis ST. Fibrin promotes migration in a three-dimensional in vitro model of wound regeneration. Tissue Eng. 2002 Oct;8(5):787-98. doi: 10.1089/10763270260424141. PMID: 12459057.
Costa KD, Lee EJ, Holmes JW. Creating alignment and anisotropy in engineered heart tissue: role of boundary conditions in a model three-dimensional culture system. Tissue Eng. 2003 Aug;9(4):567-77. doi: 10.1089/107632703768247278. PMID: 13678436.
Kuhn MA, Smith PD, Hill DP, Ko F, Meltzer DD, Vande Berg JS, Robson MC. In vitro fibroblast populated collagen lattices are not good models of in vivo clinical wound healing. Wound Repair Regen. 2000 Jul-Aug;8(4):270-6. doi: 10.1046/j.1524-475x.2000.00270.x. PMID: 11013018.