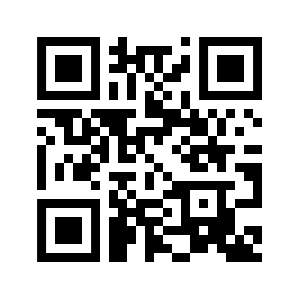
Exploring Upper Limb Kinematics in Limited Vision Conditions: Preliminary Insights from 3D Motion Analysis and IMU Data
Neurology Rehabilitation MedicinePsychiatry受け取った 20 Dec 2023 受け入れられた 17 Jan 2024 オンラインで公開された 18 Jan 2024
科学、技術、工学、医学(STEM)分野に焦点を当てています | ISSN: 2995-8067 G o o g l e Scholar
Next Full Text
The Contribution of Medical Periodicals to the Development of Pediatric Science in Modern Conditions
受け取った 20 Dec 2023 受け入れられた 17 Jan 2024 オンラインで公開された 18 Jan 2024
This study examined upper limb kinematics under simulated visually impaired conditions. By investigating how upper limb movements adapt in these circumstances, we aimed to gain insights that may prove valuable for both healthy populations and those with clinical conditions. Data, including 3D marker-based motion capture and accelerometer readings, were collected from seven healthy volunteers walking on a treadmill at 1.5 m/s under two conditions: without glasses (NG) and with stroboscopic glasses (G). Each walking condition lasted 2 minutes, and two 10-second recordings were analyzed from the midpoint of each session. Contrary to our hypothesis, simulated visual impairment with stroboscopic eyewear at 6Hz did not statistically affect upper limb kinematics, specifically in terms of wrist acceleration and hand distance in the frontal plane. Future research should explore varied visual impairment conditions, extend task durations, and investigate the relationship between subjective gait difficulties and biomechanical data.
Human gait, a complex interplay of movements, is integral to daily functioning and relies on the coordination of various body segments. While research has traditionally emphasized the analysis of lower limb biomechanics, the role of upper limb movements, especially in challenging conditions (e.g. visual impairment, pregnancy) is increasingly recognized [
, ]. The upper limbs contribute significantly to maintaining balance, stability, and adaptive movements during gait [ ]. Notably, understanding the intricate role of arm movements during gait is essential for a thorough comprehension of gait deviations, particularly in pathological gait. This significance is underscored by a substantial body of literature [ - ]. A review by Meyns, et al. [ ] examined the intricacies of arm swings during human walking, concluding that arm movements serve a dual purpose: reducing the energetic cost of gait by approximately 8% and facilitating leg movements.In central neurological pathologies like spinal cord injury, Parkinson’s disease, stroke, and Cerebral Palsy (CP), arm movements are frequently directly affected [
- ]. Despite this, the available descriptive data on upper limb movement during gait in clinical populations is limited. It has been shown that upper limb movements, including both arms and thorax movements, play a distinctive role for CP patients, varying based on the disease type, Hemiplegic (HE) or Diplegic (DI) [ , ]. CP patients adeptly use upper limb movements to compensate for physical impairments, either enhancing gait speed or mitigating altered movements on the affected side [ , ]. This underscores the importance of thoroughly investigating upper limb kinematics in populations with gait-related pathologies.Building upon this recognition, our study sought to extend this understanding to healthy individuals walking under the paradigm of limited vision conditions. By investigating how upper limb movements adapt in these circumstances, we aim to gain insights that may prove valuable for both healthy populations and those with clinical conditions, such as CP. This approach is intended not only to deepen our comprehension of walking mechanics but also to lay the groundwork for future research that could directly benefit rehabilitation strategies and assistive technology development in clinical populations.
To achieve our research objective, we employed 3D motion analysis, known for its precision in capturing intricate movement patterns [
- ]. The procedure involved placing markers on each wrist, combined with the integration of Inertial Measurement Unit (IMU) sensor data. The interplay between IMU and 3D gait data served as a crucial facet of our methodology, contributing valuable insights into the adaptations of upper limb kinematics during gait under simulated visual impairment conditions. Recent technological advancements have introduced various equipment in the field of biomechanics, enabling the measurement of motion and inertial forces during human movement [ - ]. Among these, IMUs have emerged as a widely used technology, often employed in conjunction with magnetic field sensors (MIMUs) [ - ]. IMUs, containing tri-dimensional linear accelerometers, gyroscopes, and magnetometers, are wireless sensors with a working principle based on inertia [ - ]. IMUs can function as standalone tools or be integrated into movement analysis systems, providing detailed linear and angular motion measures for individual segments, such as the head, thorax, and tibia [ - ]. The IMU sensor, placed on the wrist in our study, captured accelerations in three-dimensional space during the gait cycle. By synchronizing IMU data with the precise spatial tracking facilitated by the motion analysis, our methodology allowed a detailed investigation of the interrelationship between spatial and dynamic aspects of upper limb movements. This integrative approach offered insights into how acceleration patterns influence the intricate adjustments in upper limb kinematics observed during gait under simulated visual impairment conditions.Moreover, the induction of visually impaired scenarios was achieved through the application of stroboscopic eyewear [
]. Stroboscopic scene illumination, using brief, intense light flashes, uniquely challenges visual perception by creating the illusion of slowed or still objects [ ]. This method offers valuable insights into how the brain processes rapidly changing stimuli, making it a versatile tool for studying motion perception and temporal aspects of visual processing [ ]. The selection of stroboscopic illumination as the method to induce visual impairment was driven by its capability to replicate intermittent visual input, closely mirroring real-world situations involving limited vision [ ]. Stroboscopic eyewear, characterized by its intermittent blackouts, poses a distinctive challenge to the visual system, compelling individuals to rely on proprioceptive and vestibular inputs [ , ]. While various methods for simulating visual impairment are available, the use of stroboscopic glasses offers the advantage of precise control over the timing and duration of visual disruption, establishing a standardized and reproducible experimental setup [ ]. This specific characteristic makes it an ideal tool for investigating upper limb kinematics in a controlled environment, as it directly disrupts the visual feedback loop during gait.Our hypothesis posited that, in the presence of impaired vision, there would be a statistically significant increase in the distance between upper limb markers and wrist acceleration during walking. This expectation is based on the understanding that individuals, when faced with visual impairment, might depend more extensively on upper limb movements to preserve balance and spatial orientation.
Data encompassing both 3D marker-based motion capture and accelerometer readings were collected from seven volunteers (five males and two females, mean ± SD: age = 22.1 ± 1.7 years, height = 175.0 ± 8.6 cm, mass = 78.5 ± 9.1 kg). All participants were healthy, moderately active physical education students, and free of any known neurological or musculoskeletal disorders that could affect gait and potentially impact the expected or collected data. All procedures were conducted according to the Declaration of Helsinki and were approved by the University of Thessaly ethics committee (protocol code 2166 and date of approval 5/4/2023).
Participants walked on a treadmill (Technogym, Italy) at a constant speed of 1.5 m/s. Each participant underwent a familiarization session to acclimate to the treadmill walking. The experiment was conducted under two conditions: walking without glasses (NG) and walking with stroboscopic glasses (Nike Vapor Strobe Eyewear®), set at a 6Hz frequency (G). Each walking condition lasted for two minutes, and two 10-second recordings were analyzed from the midpoint of each session.
To capture detailed kinematic data, our study utilized 3D motion analysis. One reflective marker was placed on each wrist of the participants, facilitating precise tracking of hand movements. Additionally, an Inertial Measurement Unit (IMU) sensor was affixed to the right wrist of each participant to record acceleration data. This comprehensive setup was designed to provide an in-depth understanding of upper limb movements, particularly under conditions simulating visual impairment.
Data capture was executed, as previously described [
- ], using a Vicon Motion Capture System (Vicon T-series, Oxford, UK), which comprised ten cameras operating at a sampling rate of 100 Hz. The accelerometer data were obtained at the same frequency using a custom-made prototype accelerometer, developed as part of the “CP-WATCHER” research project under the call RESEARCH–CREATE–INNOVATE (project code: T2EDK-00759). This accelerometer was specifically designed to yield high-precision readings pertinent to our study objectives.The key variables of interest in our analysis were the hand distance in the frontal plane (HD) and the average peak Resultant Acceleration (RA) measured by the accelerometer.
The statistical analysis utilized IBM Statistics Software Package version 26 (IBM Corp., Armonk, NY). The normal distribution of HD and RA parameters was assessed using a Shapiro–Wilk test, revealing a significant difference (p < 0.05) suggesting nonnormal distribution. A Mann-Whitney U test was used for statistical analysis. The significance level was set at p < 0.05.
As depicted in Figure 1, the Mann-Whitney U test revealed no significant difference in RA between the two conditions (U = 22.0, p = 0.5887). Similarly, no significant difference in HD in the frontal plane between conditions G and NG (U = 25, p =1.0).
Contrary to our initial expectations, this study found that a visually impaired scenario, simulated using stroboscopic eyewear at a 6Hz frequency, did not notably affect upper limb kinematics, specifically in terms of wrist acceleration and hand distance in the frontal plane. This suggests that, within the scope of this experiment, healthy individuals might not significantly adjust their upper limb movements to maintain balance and orientation under mild visual impairment.
These results are in line with previous research, which suggests that the primary function of arm swing in human gait might not be directly related to enhancing stability. Instead, it could be more about aiding recovery movements, which contribute to overall gait stability [
]. However, the notable variability observed among individuals (Figure 2) in our study hints that the impact of visual impairment on gait dynamics could be more significant or subtle in different individuals or under varying conditions [ , ].The level of visual impairment introduced in our study, characterized by the use of stroboscopic eyewear, may not have been sufficiently challenging to provoke marked adaptive responses in healthy participants. This finding highlights the need for future research to examine a broader spectrum of visual impairment intensities, potentially including more severe scenarios, to thoroughly assess their influence on gait dynamics.
Several participants noted an increase in perceived difficulty towards the end of the 2-minute walking task. However, this reported increase in challenge was not reflected in the kinematic data, underscoring the complexities in correlating subjective experiences with objective biomechanical measurements. This discrepancy suggests that future studies might benefit from including longer walking durations or varied task complexities to better capture these subjective assessments.
Moreover, the study’s limitations include a small sample size and the specificity of the visual impairment simulation. Further research with a larger and more diverse sample, along with varying degrees of visual impairment, is necessary to generalize these findings. Additionally, exploring other aspects of upper limb kinematics and incorporating different walking speeds could provide a more comprehensive understanding of gait adaptations.
It is worth noting that our findings could have significant implications in clinical settings, particularly in the early detection of pathological conditions affecting gait kinematics. Wearable technology, such as smartwatch-embedded accelerometers, holds considerable promise in this regard [
, ]. Although our study did not detect these changes at a group level, refining methodologies and gathering larger datasets could enable the detection of changes at an individual level. This capability could be instrumental in assisting healthcare practitioners in identifying early stages of neurological conditions, potentially leading to timely interventions that could decelerate disease progression. This potential application highlights the increasing importance of incorporating advanced technology in clinical settings for proactive health management.In conclusion, while our study sheds light on the effects of mild visual impairment on upper limb kinematics during gait, it also underscores the necessity for additional research. Future investigations should explore a wider range of visual impairment conditions, extend task durations, and delve into the relationship between subjective gait difficulties and objective biomechanical data. Such research could provide a more holistic understanding of gait adaptations and guide the development of specialized rehabilitation strategies and assistive technologies for various populations.
This research has been co-financed by the European Regional Development Fund of the European Union and Greek national funds through the Operational Program Competitiveness, Entrepreneurship and Innovation, under the call RESEARCH–CREATE–INNOVATE (project code: Τ2ΕDΚ-00759).
Music HE, Bailey JP, Catena RD. Upper extremity kinematics during walking gait changes through pregnancy. Gait Posture. 2023 Jul; 104:97-102. doi: 10.1016/j.gaitpost.2023.06.017. Epub 2023 Jun 22. PMID: 37356228.
Sankako AN, Lucareli PRG, De Carvalho SMR, Braccialli LMP. Analysis of the positioning of the head, trunk, and upper limbs during gait in children with visual impairment. International Journal on Disability and Human Development. 2015; 14: 37-43. doi:10.1515/ijdhd-2013-0040.
Matuszewska A, Syczewska M. Analysis of the movements of the upper extremities during gait: Their role for the dynamic balance. Gait Posture. 2023 Feb; 100:82-90. doi: 10.1016/j.gaitpost.2022.12.004. Epub 2022 Dec 6. PMID: 36502665.
Bonnefoy-Mazure A, Sagawa Y Jr, Lascombes P, De Coulon G, Armand S. A descriptive analysis of the upper limb patterns during gait in individuals with cerebral palsy. Res Dev Disabil. 2014 Nov;35(11):2756-65. doi: 10.1016/j.ridd.2014.07.013. Epub 2014 Jul 31. PMID: 25084472.
Kahn MB, Williams G, Mentiplay BF, Bower KJ, Olver J, Clark RA. Quantification of abnormal upper limb movement during walking in people with acquired brain injury. Gait Posture. 2020 Sep; 81:273-280. doi: 10.1016/j.gaitpost.2020.08.110. Epub 2020 Aug 11. PMID: 32854069.
Meyns P, Desloovere K, Van Gestel L, Massaad F, Smits-Engelsman B, Duysens J. Altered arm posture in children with cerebral palsy is related to instability during walking. Eur J Paediatr Neurol. 2012 Sep;16(5):528-35. doi: 10.1016/j.ejpn.2012.01.011. Epub 2012 Feb 14. PMID: 22336190.
Sidiropoulos A, Magill R, Gordon A. Coordination of the upper and lower extremities during walking in children with cerebral palsy. Gait Posture. 2021 May; 86:251-255. doi: 10.1016/j.gaitpost.2021.03.028. Epub 2021 Mar 24. PMID: 33812293.
Hung YC, Shirzad F, Saleem M, Gordon AM. Intensive upper extremity training improved whole body movement control for children with unilateral spastic cerebral palsy. Gait Posture. 2020 Sep; 81:67-72. doi: 10.1016/j.gaitpost.2020.07.009. Epub 2020 Jul 9. PMID: 32683215; PMCID: PMC10656814.
Qin W, Yang M, Li F, Chen C, Zhen L, Tian S. Influence of positional changes on spasticity of the upper extremity in poststroke hemiplegic patients. Neurosci Lett. 2019 Nov 1; 712:134479. doi: 10.1016/j.neulet.2019.134479. Epub 2019 Sep 3. PMID: 31491464.
Meyns P, Bruijn SM, Duysens J. The how and why of arm swing during human walking. Gait Posture. 2013 Sep;38(4):555-62. doi: 10.1016/j.gaitpost.2013.02.006. Epub 2013 Mar 13. PMID: 23489950.
Ford MP, Wagenaar RC, Newell KM. Phase manipulation and walking in stroke. J Neurol Phys Ther. 2007 Jun;31(2):85-91. doi: 10.1097/NPT.0b013e3180674d18. PMID: 17558362.
Huang X, Mahoney JM, Lewis MM, Guangwei Du, Piazza SJ, Cusumano JP. Both coordination and symmetry of arm swing are reduced in Parkinson's disease. Gait Posture. 2012 Mar;35(3):373-7. doi: 10.1016/j.gaitpost.2011.10.180. Epub 2011 Nov 17. PMID: 22098825; PMCID: PMC3297736.
Ingram LA, Butler AA, Lord SR, Gandevia SC. Use of a physiological profile to document upper limb motor impairment in ageing and in neurological conditions. J Physiol. 2023 Jun;601(12):2251-2262. doi: 10.1113/JP283703. Epub 2022 Nov 7. PMID: 36271625.
Mainka S, Lauermann M, Ebersbach G. Arm swing deviations in patients with Parkinson's disease at different gait velocities. J Neural Transm (Vienna). 2023 May;130(5):655-661. doi: 10.1007/s00702-023-02619-4. Epub 2023 Mar 14. Erratum in: J Neural Transm (Vienna). 2023 May 15; PMID: 36917345; PMCID: PMC10121495.
Goudriaan M, Jonkers I, van Dieen JH, Bruijn SM. Arm swing in human walking: what is their drive? Gait Posture. 2014 Jun;40(2):321-6. doi: 10.1016/j.gaitpost.2014.04.204. Epub 2014 May 6. PMID: 24865637.
van der Kruk E, Reijne MM. Accuracy of human motion capture systems for sport applications; state-of-the-art review. Eur J Sport Sci. 2018 Jul;18(6):806-819. doi: 10.1080/17461391.2018.1463397. Epub 2018 May 9. PMID: 29741985.
Menolotto M, Komaris DS, Tedesco S, O'Flynn B, Walsh M. Motion Capture Technology in Industrial Applications: A Systematic Review. Sensors (Basel). 2020 Oct 5;20(19):5687. doi: 10.3390/s20195687. PMID: 33028042; PMCID: PMC7583783.
Topley M, Richards JG. A comparison of currently available optoelectronic motion capture systems. J Biomech. 2020 Jun 9; 106:109820. doi: 10.1016/j.jbiomech.2020.109820. Epub 2020 Apr 25. PMID: 32517978.
Adesida Y, Papi E, McGregor AH. Exploring the Role of Wearable Technology in Sport Kinematics and Kinetics: A Systematic Review. Sensors (Basel). 2019 Apr 2;19(7):1597. doi: 10.3390/s19071597. PMID: 30987014; PMCID: PMC6480145.
Aroganam G, Manivannan N, Harrison D. Review on Wearable Technology Sensors Used in Consumer Sport Applications. Sensors (Basel). 2019 Apr 28;19(9):1983. doi: 10.3390/s19091983. PMID: 31035333; PMCID: PMC6540270.
John Dian F, Vahidnia R, Rahmati A. Wearables and the Internet of Things (IoT), Applications, Opportunities, and Challenges: A Survey. IEEE Access. 2020; 8: 69200-69211. doi:10.1109/ACCESS.2020.2986329.
Lutz J, Memmert D, Raabe D, Dornberger R, Donath L. Wearables for integrative performance and tactic analyses: Opportunities, challenges, and future directions. International Journal of Environmental Research and Public Health. 2020; 17. doi:10.3390/ijerph17010059.
Pasquale DEG, Ruggeri V. Sensing strategies in wearable bio-mechanical systems for medicine and sport: A review. Journal of Micromechanics and Microengineering. 2019; 29. doi:10.1088/1361-6439/ab2f24.
Ray T, Choi J, Reeder J, Lee SP, Aranyosi AJ, Ghaffari R, Rogers JA. Soft, skin-interfaced wearable systems for sports science and analytics. Current Opinion in Biomedical Engineering. 2019; 9: 47-56. doi:10.1016/j.cobme.2019.01.003.
Rana M, Mittal V. Wearable Sensors for Real-Time Kinematics Analysis in Sports: A Review. IEEE Sensors Journal. 2021; 21: 1187-1207. doi:10.1109/JSEN.2020.3019016.
Weygers I, Kok M, Konings M, Hallez H, De Vroey H, Claeys K. Inertial Sensor-Based Lower Limb Joint Kinematics: A Methodological Systematic Review. Sensors (Basel). 2020 Jan 26;20(3):673. doi: 10.3390/s20030673. PMID: 31991862; PMCID: PMC7038336.
Piromalis DD, Kokkotis C, Tsatalas T, Bellis G, Tsaopoulos D, Zikos P, Tsotsolas N, Pizanias S, Kounelis M, Hliaoutakis A. Commercially available sensor-based monitoring and support systems in parkinson's disease: An overview. In Proceedings of the Proceedings of the 2021 8th International Conference on Computing for Sustainable Global Development. INDIACom. 2021; 430-438.
Picerno P. 25 years of lower limb joint kinematics by using inertial and magnetic sensors: A review of methodological approaches. Gait Posture. 2017 Jan; 51:239-246. doi: 10.1016/j.gaitpost.2016.11.008. Epub 2016 Nov 5. PMID: 27833057.
Ettelt D, Rey P, Jourdan G, Walther A, Robert P, Delamare J. 3D magnetic field sensor concept for use in inertial measurement units (IMUs). Journal of Microelectromechanical Systems. 2014; 23: 324-333. doi:10.1109/JMEMS.2013.2273362.
Teufl W, Miezal M, Taetz B, Fröhlich M, Bleser G. Validity, Test-Retest Reliability and Long-Term Stability of Magnetometer Free Inertial Sensor Based 3D Joint Kinematics. Sensors (Basel). 2018 Jun 21;18(7):1980. doi: 10.3390/s18071980. PMID: 29933568; PMCID: PMC6068643.
Ribeiro NF, Santos CP. Inertial measurement units: A brief state of the art on gait analysis. In Proceedings of the ENBENG 2017 - 5th Portuguese Meeting on Bioengineering. Proceedings. 2017.
Digo E, Gastaldi L, Antonelli M, Pastorelli S, Cereatti A, Caruso M. Real-time estimation of upper limbs kinematics with IMUs during typical industrial gestures. In Proceedings of the Procedia Computer Science. 2022; 1041-1047.
Fong DT, Chan YY. The use of wearable inertial motion sensors in human lower limb biomechanics studies: a systematic review. Sensors (Basel). 2010;10(12):11556-65. doi: 10.3390/s101211556. Epub 2010 Dec 16. PMID: 22163542; PMCID: PMC3231075.
Seel T, Raisch J, Schauer T. IMU-based joint angle measurement for gait analysis. Sensors (Basel). 2014 Apr 16;14(4):6891-909. doi: 10.3390/s140406891. PMID: 24743160; PMCID: PMC4029684.
O'Reilly M, Caulfield B, Ward T, Johnston W, Doherty C. Wearable Inertial Sensor Systems for Lower Limb Exercise Detection and Evaluation: A Systematic Review. Sports Med. 2018 May;48(5):1221-1246. doi: 10.1007/s40279-018-0878-4. PMID: 29476427.
Teufl W, Miezal M, Taetz B, Fröhlich M, Bleser G. Validity of inertial sensor based 3D joint kinematics of static and dynamic sport and physiotherapy specific movements. PLoS One. 2019 Feb 28;14(2):e0213064. doi: 10.1371/journal.pone.0213064. PMID: 30817787; PMCID: PMC6394915.
Das J, Walker R, Barry G, Vitório R, Stuart S, Morris R. Stroboscopic visual training: The potential for clinical application in neurological populations. PLOS Digit Health. 2023 Aug 23;2(8):e0000335. doi: 10.1371/journal.pdig.0000335. PMID: 37611053; PMCID: PMC10446176.
Hülsdünker T, Fontaine G, Mierau A. Stroboscopic vision prolongs visual motion perception in the central nervous system. Scand J Med Sci Sports. 2023 Jan;33(1):47-54. doi: 10.1111/sms.14239. Epub 2022 Sep 23. PMID: 36111383.
Kim KM, Kim JS, Oh J, Grooms DR. Stroboscopic vision as a dynamic sensory reweighting alternative to the sensory organization test. Journal of Sport Rehabilitation. 2021; 30: 166-172. doi:10.1123/jsr.2019-0466.
Appelbaum LG, Schroeder JE, Cain MS, Mitroff SR. Improved Visual Cognition through Stroboscopic Training. Front Psychol. 2011 Oct 28; 2:276. doi: 10.3389/fpsyg.2011.00276. PMID: 22059078; PMCID: PMC3203550.
Wilkins L, Appelbaum LG. An early review of stroboscopic visual training: insights, challenges and accomplishments to guide future studies. International Review of Sport and Exercise Psychology. 2020; 13: 65-80. doi:10.1080/1750984X.2019.1582081.
Symeonidou ER, Ferris DP. Intermittent Visual Occlusions Increase Balance Training Effectiveness. Front Hum Neurosci. 2022 Apr 25; 16:748930. doi: 10.3389/fnhum.2022.748930. PMID: 35547194; PMCID: PMC9083907.
Kokkotis C, Moustakidis S, Tsatalas T, Ntakolia C, Chalatsis G, Konstadakos S, Hantes ME, Giakas G, Tsaopoulos D. Leveraging explainable machine learning to identify gait biomechanical parameters associated with anterior cruciate ligament injury. Sci Rep. 2022 Apr 22;12(1):6647. doi: 10.1038/s41598-022-10666-2. PMID: 35459787; PMCID: PMC9026057.
Tsatalas T, Giakas G, Spyropoulos G, Sideris V, Kotzamanidis C, Koutedakis Y. Walking kinematics and kinetics following eccentric exercise-induced muscle damage. J Electromyogr Kinesiol. 2013 Oct;23(5):1229-36. doi: 10.1016/j.jelekin.2013.04.008. Epub 2013 May 17. PMID: 23688777.
Tsatalas T, Karampina E, Mina MA, Patikas DA, Laschou VC, Pappas A, Jamurtas AZ, Koutedakis Y, Giakas G. Altered Drop Jump Landing Biomechanics Following Eccentric Exercise-Induced Muscle Damage. Sports (Basel). 2021 Feb 5;9(2):24. doi: 10.3390/sports9020024. PMID: 33562760; PMCID: PMC7915566.
Bruijn SM, Meijer OG, Beek PJ, van Dieën JH. The effects of arm swing on human gait stability. J Exp Biol. 2010 Dec 1;213(Pt 23):3945-52. doi: 10.1242/jeb.045112. PMID: 21075935.
Clark-Carter DD, Heyes AD, Howarth CI. The efficiency and walking speed of visually impaired people. Ergonomics. 1986 Jun;29(6):779-89. doi: 10.1080/00140138608968314. PMID: 3743536.
Hallemans A, Ortibus E, Meire F, Aerts P. Low vision affects dynamic stability of gait. Gait Posture. 2010 Oct;32(4):547-51. doi: 10.1016/j.gaitpost.2010.07.018. PMID: 20801658.
Piromalis D, Kounelis M, Kolovos DP, Kokkotis C, Tsatalas T, Bellis G, Tsaopoulos D, Giakas G, Chronakis A, Koutsouraki E. Portable gait analysis sensor model for Parkinson's disease. Materials Today: Proceedings. 2022; 63: 653-662. doi:10.1016/j.matpr.2022.04.724.
Zarkadoula A, Tsatalas T, Bellis G, Papaggelos P, Vlahogianni E, Moustos S, Koukourava E, Tsaopoulos D. Exploring Upper Limb Kinematics in Limited Vision Conditions: Preliminary Insights from 3D Motion Analysis and IMU Data. IgMin Res. 18 Jan, 2024; 2(1): 018-022. IgMin ID: igmin138; DOI: 10.61927/igmin138; Available at: www.igminresearch.com/articles/pdf/igmin138.pdf
次のリンクを共有した人は、このコンテンツを読むことができます:
1DPESS, University of Thessaly, Trikala, Greece
1,2DPESS, University of Thessaly, Trikala, Greece | Biomechanical Solutions, Karditsa, Greece
2Biomechanical Solutions, Karditsa, Greece
2Biomechanical Solutions, Karditsa, Greece
2Biomechanical Solutions, Karditsa, Greece
2Biomechanical Solutions, Karditsa, Greece
3Animus Rehabilitation Centre, Larisa, Greece
4IBO, CERTH, Thessaloniki, Greece
Address Correspondence:
Themistoklis Tsatalas, PhD, DPESS, University of Thessaly, Trikala, Biomechanical Solutions, Karditsa, Greece, Email: ttsatalas@uth.gr
How to cite this article:
Zarkadoula A, Tsatalas T, Bellis G, Papaggelos P, Vlahogianni E, Moustos S, Koukourava E, Tsaopoulos D. Exploring Upper Limb Kinematics in Limited Vision Conditions: Preliminary Insights from 3D Motion Analysis and IMU Data. IgMin Res. 18 Jan, 2024; 2(1): 018-022. IgMin ID: igmin138; DOI: 10.61927/igmin138; Available at: www.igminresearch.com/articles/pdf/igmin138.pdf
Copyright: © 2024 Zarkadoula A, et al. This is an open access article distributed under the Creative Commons Attribution License, which permits unrestricted use, distribution, and reproduction in any medium, provided the original work is properly cited.
Music HE, Bailey JP, Catena RD. Upper extremity kinematics during walking gait changes through pregnancy. Gait Posture. 2023 Jul; 104:97-102. doi: 10.1016/j.gaitpost.2023.06.017. Epub 2023 Jun 22. PMID: 37356228.
Sankako AN, Lucareli PRG, De Carvalho SMR, Braccialli LMP. Analysis of the positioning of the head, trunk, and upper limbs during gait in children with visual impairment. International Journal on Disability and Human Development. 2015; 14: 37-43. doi:10.1515/ijdhd-2013-0040.
Matuszewska A, Syczewska M. Analysis of the movements of the upper extremities during gait: Their role for the dynamic balance. Gait Posture. 2023 Feb; 100:82-90. doi: 10.1016/j.gaitpost.2022.12.004. Epub 2022 Dec 6. PMID: 36502665.
Bonnefoy-Mazure A, Sagawa Y Jr, Lascombes P, De Coulon G, Armand S. A descriptive analysis of the upper limb patterns during gait in individuals with cerebral palsy. Res Dev Disabil. 2014 Nov;35(11):2756-65. doi: 10.1016/j.ridd.2014.07.013. Epub 2014 Jul 31. PMID: 25084472.
Kahn MB, Williams G, Mentiplay BF, Bower KJ, Olver J, Clark RA. Quantification of abnormal upper limb movement during walking in people with acquired brain injury. Gait Posture. 2020 Sep; 81:273-280. doi: 10.1016/j.gaitpost.2020.08.110. Epub 2020 Aug 11. PMID: 32854069.
Meyns P, Desloovere K, Van Gestel L, Massaad F, Smits-Engelsman B, Duysens J. Altered arm posture in children with cerebral palsy is related to instability during walking. Eur J Paediatr Neurol. 2012 Sep;16(5):528-35. doi: 10.1016/j.ejpn.2012.01.011. Epub 2012 Feb 14. PMID: 22336190.
Sidiropoulos A, Magill R, Gordon A. Coordination of the upper and lower extremities during walking in children with cerebral palsy. Gait Posture. 2021 May; 86:251-255. doi: 10.1016/j.gaitpost.2021.03.028. Epub 2021 Mar 24. PMID: 33812293.
Hung YC, Shirzad F, Saleem M, Gordon AM. Intensive upper extremity training improved whole body movement control for children with unilateral spastic cerebral palsy. Gait Posture. 2020 Sep; 81:67-72. doi: 10.1016/j.gaitpost.2020.07.009. Epub 2020 Jul 9. PMID: 32683215; PMCID: PMC10656814.
Qin W, Yang M, Li F, Chen C, Zhen L, Tian S. Influence of positional changes on spasticity of the upper extremity in poststroke hemiplegic patients. Neurosci Lett. 2019 Nov 1; 712:134479. doi: 10.1016/j.neulet.2019.134479. Epub 2019 Sep 3. PMID: 31491464.
Meyns P, Bruijn SM, Duysens J. The how and why of arm swing during human walking. Gait Posture. 2013 Sep;38(4):555-62. doi: 10.1016/j.gaitpost.2013.02.006. Epub 2013 Mar 13. PMID: 23489950.
Ford MP, Wagenaar RC, Newell KM. Phase manipulation and walking in stroke. J Neurol Phys Ther. 2007 Jun;31(2):85-91. doi: 10.1097/NPT.0b013e3180674d18. PMID: 17558362.
Huang X, Mahoney JM, Lewis MM, Guangwei Du, Piazza SJ, Cusumano JP. Both coordination and symmetry of arm swing are reduced in Parkinson's disease. Gait Posture. 2012 Mar;35(3):373-7. doi: 10.1016/j.gaitpost.2011.10.180. Epub 2011 Nov 17. PMID: 22098825; PMCID: PMC3297736.
Ingram LA, Butler AA, Lord SR, Gandevia SC. Use of a physiological profile to document upper limb motor impairment in ageing and in neurological conditions. J Physiol. 2023 Jun;601(12):2251-2262. doi: 10.1113/JP283703. Epub 2022 Nov 7. PMID: 36271625.
Mainka S, Lauermann M, Ebersbach G. Arm swing deviations in patients with Parkinson's disease at different gait velocities. J Neural Transm (Vienna). 2023 May;130(5):655-661. doi: 10.1007/s00702-023-02619-4. Epub 2023 Mar 14. Erratum in: J Neural Transm (Vienna). 2023 May 15; PMID: 36917345; PMCID: PMC10121495.
Goudriaan M, Jonkers I, van Dieen JH, Bruijn SM. Arm swing in human walking: what is their drive? Gait Posture. 2014 Jun;40(2):321-6. doi: 10.1016/j.gaitpost.2014.04.204. Epub 2014 May 6. PMID: 24865637.
van der Kruk E, Reijne MM. Accuracy of human motion capture systems for sport applications; state-of-the-art review. Eur J Sport Sci. 2018 Jul;18(6):806-819. doi: 10.1080/17461391.2018.1463397. Epub 2018 May 9. PMID: 29741985.
Menolotto M, Komaris DS, Tedesco S, O'Flynn B, Walsh M. Motion Capture Technology in Industrial Applications: A Systematic Review. Sensors (Basel). 2020 Oct 5;20(19):5687. doi: 10.3390/s20195687. PMID: 33028042; PMCID: PMC7583783.
Topley M, Richards JG. A comparison of currently available optoelectronic motion capture systems. J Biomech. 2020 Jun 9; 106:109820. doi: 10.1016/j.jbiomech.2020.109820. Epub 2020 Apr 25. PMID: 32517978.
Adesida Y, Papi E, McGregor AH. Exploring the Role of Wearable Technology in Sport Kinematics and Kinetics: A Systematic Review. Sensors (Basel). 2019 Apr 2;19(7):1597. doi: 10.3390/s19071597. PMID: 30987014; PMCID: PMC6480145.
Aroganam G, Manivannan N, Harrison D. Review on Wearable Technology Sensors Used in Consumer Sport Applications. Sensors (Basel). 2019 Apr 28;19(9):1983. doi: 10.3390/s19091983. PMID: 31035333; PMCID: PMC6540270.
John Dian F, Vahidnia R, Rahmati A. Wearables and the Internet of Things (IoT), Applications, Opportunities, and Challenges: A Survey. IEEE Access. 2020; 8: 69200-69211. doi:10.1109/ACCESS.2020.2986329.
Lutz J, Memmert D, Raabe D, Dornberger R, Donath L. Wearables for integrative performance and tactic analyses: Opportunities, challenges, and future directions. International Journal of Environmental Research and Public Health. 2020; 17. doi:10.3390/ijerph17010059.
Pasquale DEG, Ruggeri V. Sensing strategies in wearable bio-mechanical systems for medicine and sport: A review. Journal of Micromechanics and Microengineering. 2019; 29. doi:10.1088/1361-6439/ab2f24.
Ray T, Choi J, Reeder J, Lee SP, Aranyosi AJ, Ghaffari R, Rogers JA. Soft, skin-interfaced wearable systems for sports science and analytics. Current Opinion in Biomedical Engineering. 2019; 9: 47-56. doi:10.1016/j.cobme.2019.01.003.
Rana M, Mittal V. Wearable Sensors for Real-Time Kinematics Analysis in Sports: A Review. IEEE Sensors Journal. 2021; 21: 1187-1207. doi:10.1109/JSEN.2020.3019016.
Weygers I, Kok M, Konings M, Hallez H, De Vroey H, Claeys K. Inertial Sensor-Based Lower Limb Joint Kinematics: A Methodological Systematic Review. Sensors (Basel). 2020 Jan 26;20(3):673. doi: 10.3390/s20030673. PMID: 31991862; PMCID: PMC7038336.
Piromalis DD, Kokkotis C, Tsatalas T, Bellis G, Tsaopoulos D, Zikos P, Tsotsolas N, Pizanias S, Kounelis M, Hliaoutakis A. Commercially available sensor-based monitoring and support systems in parkinson's disease: An overview. In Proceedings of the Proceedings of the 2021 8th International Conference on Computing for Sustainable Global Development. INDIACom. 2021; 430-438.
Picerno P. 25 years of lower limb joint kinematics by using inertial and magnetic sensors: A review of methodological approaches. Gait Posture. 2017 Jan; 51:239-246. doi: 10.1016/j.gaitpost.2016.11.008. Epub 2016 Nov 5. PMID: 27833057.
Ettelt D, Rey P, Jourdan G, Walther A, Robert P, Delamare J. 3D magnetic field sensor concept for use in inertial measurement units (IMUs). Journal of Microelectromechanical Systems. 2014; 23: 324-333. doi:10.1109/JMEMS.2013.2273362.
Teufl W, Miezal M, Taetz B, Fröhlich M, Bleser G. Validity, Test-Retest Reliability and Long-Term Stability of Magnetometer Free Inertial Sensor Based 3D Joint Kinematics. Sensors (Basel). 2018 Jun 21;18(7):1980. doi: 10.3390/s18071980. PMID: 29933568; PMCID: PMC6068643.
Ribeiro NF, Santos CP. Inertial measurement units: A brief state of the art on gait analysis. In Proceedings of the ENBENG 2017 - 5th Portuguese Meeting on Bioengineering. Proceedings. 2017.
Digo E, Gastaldi L, Antonelli M, Pastorelli S, Cereatti A, Caruso M. Real-time estimation of upper limbs kinematics with IMUs during typical industrial gestures. In Proceedings of the Procedia Computer Science. 2022; 1041-1047.
Fong DT, Chan YY. The use of wearable inertial motion sensors in human lower limb biomechanics studies: a systematic review. Sensors (Basel). 2010;10(12):11556-65. doi: 10.3390/s101211556. Epub 2010 Dec 16. PMID: 22163542; PMCID: PMC3231075.
Seel T, Raisch J, Schauer T. IMU-based joint angle measurement for gait analysis. Sensors (Basel). 2014 Apr 16;14(4):6891-909. doi: 10.3390/s140406891. PMID: 24743160; PMCID: PMC4029684.
O'Reilly M, Caulfield B, Ward T, Johnston W, Doherty C. Wearable Inertial Sensor Systems for Lower Limb Exercise Detection and Evaluation: A Systematic Review. Sports Med. 2018 May;48(5):1221-1246. doi: 10.1007/s40279-018-0878-4. PMID: 29476427.
Teufl W, Miezal M, Taetz B, Fröhlich M, Bleser G. Validity of inertial sensor based 3D joint kinematics of static and dynamic sport and physiotherapy specific movements. PLoS One. 2019 Feb 28;14(2):e0213064. doi: 10.1371/journal.pone.0213064. PMID: 30817787; PMCID: PMC6394915.
Das J, Walker R, Barry G, Vitório R, Stuart S, Morris R. Stroboscopic visual training: The potential for clinical application in neurological populations. PLOS Digit Health. 2023 Aug 23;2(8):e0000335. doi: 10.1371/journal.pdig.0000335. PMID: 37611053; PMCID: PMC10446176.
Hülsdünker T, Fontaine G, Mierau A. Stroboscopic vision prolongs visual motion perception in the central nervous system. Scand J Med Sci Sports. 2023 Jan;33(1):47-54. doi: 10.1111/sms.14239. Epub 2022 Sep 23. PMID: 36111383.
Kim KM, Kim JS, Oh J, Grooms DR. Stroboscopic vision as a dynamic sensory reweighting alternative to the sensory organization test. Journal of Sport Rehabilitation. 2021; 30: 166-172. doi:10.1123/jsr.2019-0466.
Appelbaum LG, Schroeder JE, Cain MS, Mitroff SR. Improved Visual Cognition through Stroboscopic Training. Front Psychol. 2011 Oct 28; 2:276. doi: 10.3389/fpsyg.2011.00276. PMID: 22059078; PMCID: PMC3203550.
Wilkins L, Appelbaum LG. An early review of stroboscopic visual training: insights, challenges and accomplishments to guide future studies. International Review of Sport and Exercise Psychology. 2020; 13: 65-80. doi:10.1080/1750984X.2019.1582081.
Symeonidou ER, Ferris DP. Intermittent Visual Occlusions Increase Balance Training Effectiveness. Front Hum Neurosci. 2022 Apr 25; 16:748930. doi: 10.3389/fnhum.2022.748930. PMID: 35547194; PMCID: PMC9083907.
Kokkotis C, Moustakidis S, Tsatalas T, Ntakolia C, Chalatsis G, Konstadakos S, Hantes ME, Giakas G, Tsaopoulos D. Leveraging explainable machine learning to identify gait biomechanical parameters associated with anterior cruciate ligament injury. Sci Rep. 2022 Apr 22;12(1):6647. doi: 10.1038/s41598-022-10666-2. PMID: 35459787; PMCID: PMC9026057.
Tsatalas T, Giakas G, Spyropoulos G, Sideris V, Kotzamanidis C, Koutedakis Y. Walking kinematics and kinetics following eccentric exercise-induced muscle damage. J Electromyogr Kinesiol. 2013 Oct;23(5):1229-36. doi: 10.1016/j.jelekin.2013.04.008. Epub 2013 May 17. PMID: 23688777.
Tsatalas T, Karampina E, Mina MA, Patikas DA, Laschou VC, Pappas A, Jamurtas AZ, Koutedakis Y, Giakas G. Altered Drop Jump Landing Biomechanics Following Eccentric Exercise-Induced Muscle Damage. Sports (Basel). 2021 Feb 5;9(2):24. doi: 10.3390/sports9020024. PMID: 33562760; PMCID: PMC7915566.
Bruijn SM, Meijer OG, Beek PJ, van Dieën JH. The effects of arm swing on human gait stability. J Exp Biol. 2010 Dec 1;213(Pt 23):3945-52. doi: 10.1242/jeb.045112. PMID: 21075935.
Clark-Carter DD, Heyes AD, Howarth CI. The efficiency and walking speed of visually impaired people. Ergonomics. 1986 Jun;29(6):779-89. doi: 10.1080/00140138608968314. PMID: 3743536.
Hallemans A, Ortibus E, Meire F, Aerts P. Low vision affects dynamic stability of gait. Gait Posture. 2010 Oct;32(4):547-51. doi: 10.1016/j.gaitpost.2010.07.018. PMID: 20801658.
Piromalis D, Kounelis M, Kolovos DP, Kokkotis C, Tsatalas T, Bellis G, Tsaopoulos D, Giakas G, Chronakis A, Koutsouraki E. Portable gait analysis sensor model for Parkinson's disease. Materials Today: Proceedings. 2022; 63: 653-662. doi:10.1016/j.matpr.2022.04.724.